Unconventional translation of mammalian LINE-1 retrotransposons
- PMID: 16418485
- PMCID: PMC1356112
- DOI: 10.1101/gad.1380406
Unconventional translation of mammalian LINE-1 retrotransposons
Abstract
Long Interspersed Element-1 (LINE-1 or L1) retrotransposons encode proteins required for their mobility (ORF1p and ORF2p), yet little is known about how L1 mRNA is translated. Here, we show that ORF2 translation generally initiates from the first in-frame methionine codon of ORF2, and that both ORF1 and the inter-ORF spacer are dispensable for ORF2 translation. Remarkably, changing the ORF2 AUG codon to any other coding triplet is compatible with retrotransposition. However, introducing a premature termination codon in ORF1 or a thermostable hairpin in the inter-ORF spacer reduces ORF2p translation or L1 retrotransposition to approximately 5% of wild-type levels. Similar data obtained from "natural" and codon optimized "synthetic" mouse L1s lead us to propose that ORF2 is translated by an unconventional termination/reinitiation mechanism.
Figures
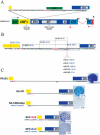
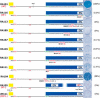
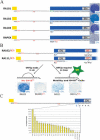
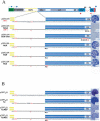
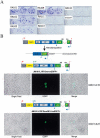
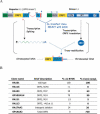
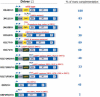
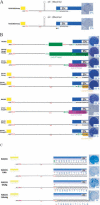
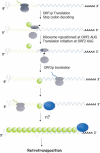
Similar articles
-
Eukaryotic translational coupling in UAAUG stop-start codons for the bicistronic RNA translation of the non-long terminal repeat retrotransposon SART1.Mol Cell Biol. 2005 Sep;25(17):7675-86. doi: 10.1128/MCB.25.17.7675-7686.2005. Mol Cell Biol. 2005. PMID: 16107714 Free PMC article.
-
Low dependency of retrotransposition on the ORF1 protein of the zebrafish LINE, ZfL2-1.Gene. 2012 May 10;499(1):41-7. doi: 10.1016/j.gene.2012.02.048. Epub 2012 Mar 3. Gene. 2012. PMID: 22405944
-
Cis-preferential LINE-1 reverse transcriptase activity in ribonucleoprotein particles.Nat Struct Mol Biol. 2006 Jul;13(7):655-60. doi: 10.1038/nsmb1107. Epub 2006 Jun 18. Nat Struct Mol Biol. 2006. PMID: 16783376
-
Nucleic acid chaperone properties of ORF1p from the non-LTR retrotransposon, LINE-1.RNA Biol. 2010 Nov-Dec;7(6):706-11. doi: 10.4161/rna.7.6.13766. Epub 2010 Nov 1. RNA Biol. 2010. PMID: 21045547 Free PMC article. Review.
-
Translational termination-reinitiation in RNA viruses.Biochem Soc Trans. 2010 Dec;38(6):1558-64. doi: 10.1042/BST0381558. Biochem Soc Trans. 2010. PMID: 21118126 Review.
Cited by
-
Retrotransposon life cycle and its impacts on cellular responses.RNA Biol. 2024 Jan;21(1):11-27. doi: 10.1080/15476286.2024.2409607. Epub 2024 Oct 13. RNA Biol. 2024. PMID: 39396200 Free PMC article. Review.
-
Variable patterns of retrotransposition in different HeLa strains provide mechanistic insights into SINE RNA mobilization processes.Nucleic Acids Res. 2024 Jul 22;52(13):7761-7779. doi: 10.1093/nar/gkae448. Nucleic Acids Res. 2024. PMID: 38850156 Free PMC article.
-
Retrotransposons in embryogenesis and neurodevelopment.Biochem Soc Trans. 2024 Jun 26;52(3):1159-1171. doi: 10.1042/BST20230757. Biochem Soc Trans. 2024. PMID: 38716891 Free PMC article. Review.
-
Celine, a long interspersed nuclear element retrotransposon, colonizes in the centromeres of poplar chromosomes.Plant Physiol. 2024 Jul 31;195(4):2787-2798. doi: 10.1093/plphys/kiae214. Plant Physiol. 2024. PMID: 38652695 Free PMC article.
-
Large Deletions, Cleavage of the Telomeric Repeat Sequence, and Reverse Transcriptase-Mediated DNA Damage Response Associated with Long Interspersed Element-1 ORF2p Enzymatic Activities.Genes (Basel). 2024 Jan 23;15(2):143. doi: 10.3390/genes15020143. Genes (Basel). 2024. PMID: 38397133 Free PMC article.
References
-
- Boissinot, S., Chevret, P., and Furano, A.V. 2000. L1 (LINE-1) retrotransposon evolution and amplification in recent human history. Mol. Biol. Evol. 17: 915-928. - PubMed
Publication types
MeSH terms
Substances
Grants and funding
LinkOut - more resources
Full Text Sources