Battles with iron: manganese in oxidative stress protection
- PMID: 22247543
- PMCID: PMC3340200
- DOI: 10.1074/jbc.R111.312181
Battles with iron: manganese in oxidative stress protection
Abstract
The redox-active metal manganese plays a key role in cellular adaptation to oxidative stress. As a cofactor for manganese superoxide dismutase or through formation of non-proteinaceous manganese antioxidants, this metal can combat oxidative damage without deleterious side effects of Fenton chemistry. In either case, the antioxidant properties of manganese are vulnerable to iron. Cellular pools of iron can outcompete manganese for binding to manganese superoxide dismutase, and through Fenton chemistry, iron may counteract the benefits of non-proteinaceous manganese antioxidants. In this minireview, we highlight ways in which cells maximize the efficacy of manganese as an antioxidant in the midst of pro-oxidant iron.
Figures
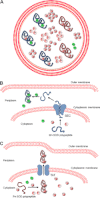
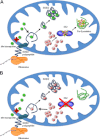
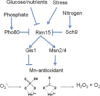
Similar articles
-
Iron, copper, and manganese complexes with in vitro superoxide dismutase and/or catalase activities that keep Saccharomyces cerevisiae cells alive under severe oxidative stress.Free Radic Biol Med. 2015 Mar;80:67-76. doi: 10.1016/j.freeradbiomed.2014.12.005. Epub 2014 Dec 13. Free Radic Biol Med. 2015. PMID: 25511255
-
Catalytic antioxidant therapy by metallodrugs: lessons from metallocorroles.Chem Commun (Camb). 2015 Apr 7;51(27):5812-27. doi: 10.1039/c4cc08715a. Epub 2015 Feb 9. Chem Commun (Camb). 2015. PMID: 25664356 Review.
-
A Single Mutation is Sufficient to Modify the Metal Selectivity and Specificity of a Eukaryotic Manganese Superoxide Dismutase to Encompass Iron.Chemistry. 2018 Apr 6;24(20):5303-5308. doi: 10.1002/chem.201704655. Epub 2017 Dec 12. Chemistry. 2018. PMID: 29178484 Free PMC article.
-
The mismetallation of enzymes during oxidative stress.J Biol Chem. 2014 Oct 10;289(41):28121-8. doi: 10.1074/jbc.R114.588814. Epub 2014 Aug 26. J Biol Chem. 2014. PMID: 25160623 Free PMC article. Review.
-
Manganese activation of superoxide dismutase 2 in the mitochondria of Saccharomyces cerevisiae.J Biol Chem. 2005 Jun 17;280(24):22715-20. doi: 10.1074/jbc.M504257200. Epub 2005 Apr 25. J Biol Chem. 2005. PMID: 15851472
Cited by
-
The Evaluation of Selected Trace Elements in Blood, Serum and Blood Cells of Type 2 Diabetes Patients with and without Renal Disorder.Nutrients. 2024 Sep 4;16(17):2989. doi: 10.3390/nu16172989. Nutrients. 2024. PMID: 39275304 Free PMC article.
-
Is oxidative stress - antioxidants imbalance the physiopathogenic core in pediatric obesity?Front Immunol. 2024 Aug 8;15:1394869. doi: 10.3389/fimmu.2024.1394869. eCollection 2024. Front Immunol. 2024. PMID: 39176098 Free PMC article. Review.
-
Exploring the _targetome of IsrR, an iron-regulated sRNA controlling the synthesis of iron-containing proteins in Staphylococcus aureus.Front Microbiol. 2024 Jul 5;15:1439352. doi: 10.3389/fmicb.2024.1439352. eCollection 2024. Front Microbiol. 2024. PMID: 39035440 Free PMC article.
-
Fluorescent Protein-Based Sensors for Detecting Essential Metal Ions across the Tree of Life.ACS Sens. 2024 Apr 26;9(4):1622-1643. doi: 10.1021/acssensors.3c02695. Epub 2024 Apr 8. ACS Sens. 2024. PMID: 38587931 Review.
-
Metal and oxidative potential exposure through particle inhalation and oxidative stress biomarkers: a 2-week pilot prospective study among Parisian subway workers.Int Arch Occup Environ Health. 2024 May;97(4):387-400. doi: 10.1007/s00420-024-02054-2. Epub 2024 Mar 20. Int Arch Occup Environ Health. 2024. PMID: 38504030 Free PMC article.
References
-
- Dupont C. L., Neupane K., Shearer J., Palenik B. (2008) Diversity, function, and evolution of genes coding for putative nickel-containing superoxide dismutases. Environ. Microbiol. 10, 1831–1843 - PubMed
-
- May B. P., Dennis P. P. (1989) Evolution and regulation of the gene encoding superoxide dismutase from the archaebacterium Halobacterium cutirubrum. J. Biol. Chem. 264, 12253–12258 - PubMed
-
- Wintjens R., Noël C., May A. C., Gerbod D., Dufernez F., Capron M., Viscogliosi E., Rooman M. (2004) Specificity and phenetic relationships of iron- and manganese-containing superoxide dismutases on the basis of structure and sequence comparisons. J. Biol. Chem. 279, 9248–9254 - PubMed
-
- Whittaker J. W. (2003) The irony of manganese superoxide dismutase. Biochem. Soc. Trans. 31, 1318–1321 - PubMed
-
- Culotta V. C., Klomp L. W., Strain J., Casareno R. L., Krems B., Gitlin J. D. (1997) The copper chaperone for superoxide dismutase. J. Biol. Chem. 272, 23469–23472 - PubMed
Publication types
MeSH terms
Substances
Grants and funding
LinkOut - more resources
Full Text Sources
Medical
Molecular Biology Databases
Research Materials