Alternative Splicing of the Pituitary Adenylate Cyclase-Activating Polypeptide Receptor PAC1: Mechanisms of Fine Tuning of Brain Activity
- PMID: 23734144
- PMCID: PMC3659299
- DOI: 10.3389/fendo.2013.00055
Alternative Splicing of the Pituitary Adenylate Cyclase-Activating Polypeptide Receptor PAC1: Mechanisms of Fine Tuning of Brain Activity
Abstract
Alternative splicing of the precursor mRNA encoding for the neuropeptide receptor PAC1/ADCYAP1R1 generates multiple protein products that exhibit pleiotropic activities. Recent studies in mammals and zebrafish have implicated some of these splice isoforms in control of both cellular and body homeostasis. Here, we review the regulation of PAC1 splice variants and their underlying signal transduction and physiological processes in the nervous system.
Keywords: ADCYAP1R1; PACAP receptor; activity-dependent gene regulation; homeostasis; hypothalamic hormones; post-traumatic; stress disorders; zebrafish model system.
Figures
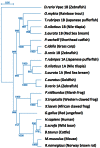

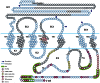
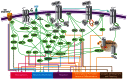
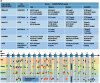
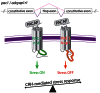
Similar articles
-
Novel splice variants of type I pituitary adenylate cyclase-activating polypeptide receptor in frog exhibit altered adenylate cyclase stimulation and differential relative abundance.Endocrinology. 2002 Jul;143(7):2680-92. doi: 10.1210/endo.143.7.8880. Endocrinology. 2002. PMID: 12072402
-
Pituitary adenylyl cyclase-activating polypeptide (PACAP) and its receptor (PAC1-R) in the cochlea: evidence for specific transcript expression of PAC1-R splice variants in rat microdissected cochlear subfractions.Neuroscience. 2006 Jun 19;140(1):147-61. doi: 10.1016/j.neuroscience.2006.01.019. Epub 2006 Apr 19. Neuroscience. 2006. PMID: 16626868
-
Pituitary adenylate cyclase-activating polypeptide (PACAP) and PACAP-receptor type 1 expression in rat and human placenta.Endocrinology. 2000 Mar;141(3):1158-67. doi: 10.1210/endo.141.3.7346. Endocrinology. 2000. PMID: 10698193
-
Pituitary adenylate cyclase-activating polypeptide (PACAP) and its receptors in the brain.Kaibogaku Zasshi. 2000 Dec;75(6):487-507. Kaibogaku Zasshi. 2000. PMID: 11197592 Review.
-
Perspectives on pituitary adenylate cyclase activating polypeptide (PACAP) in the neuroendocrine, endocrine, and nervous systems.Jpn J Physiol. 1998 Oct;48(5):301-31. doi: 10.2170/jjphysiol.48.301. Jpn J Physiol. 1998. PMID: 9852340 Review.
Cited by
-
The Role of Pituitary Adenylate Cyclase-Activating Polypeptide (PACAP) Signaling in the Hippocampal Dentate Gyrus.Front Cell Neurosci. 2020 Apr 30;14:111. doi: 10.3389/fncel.2020.00111. eCollection 2020. Front Cell Neurosci. 2020. PMID: 32425759 Free PMC article.
-
PACAP receptor pharmacology and agonist bias: analysis in primary neurons and glia from the trigeminal ganglia and transfected cells.Br J Pharmacol. 2014 Mar;171(6):1521-33. doi: 10.1111/bph.12541. Br J Pharmacol. 2014. PMID: 24303997 Free PMC article.
-
Secreted key regulators (Fgf1, Bmp4, Gdf3) are expressed by PAC1-immunopositive retinal ganglion cells in the postnatal rat retina.Eur J Histochem. 2022 Apr 27;66(2):3373. doi: 10.4081/ejh.2022.3373. Eur J Histochem. 2022. PMID: 35477223 Free PMC article.
-
New Insights into the Structure and Function of Class B1 GPCRs.Endocr Rev. 2023 May 8;44(3):492-517. doi: 10.1210/endrev/bnac033. Endocr Rev. 2023. PMID: 36546772 Free PMC article.
-
Exploring the Pro-Phagocytic and Anti-Inflammatory Functions of PACAP and VIP in Microglia: Implications for Multiple Sclerosis.Int J Mol Sci. 2022 Apr 26;23(9):4788. doi: 10.3390/ijms23094788. Int J Mol Sci. 2022. PMID: 35563181 Free PMC article. Review.
References
-
- Abu-Hamdan M. D., Drescher M. J., Ramakrishnan N. A., Khan K. M., Toma V. S., Hatfield J. S., et al. (2006). Pituitary adenylyl cyclase-activating polypeptide (PACAP) and its receptor (PAC1-R) in the cochlea: evidence for specific transcript expression of PAC1-R splice variants in rat microdissected cochlear subfractions. Neuroscience 140, 147–16110.1016/j.neuroscience.2006.01.019 - DOI - PubMed
-
- Agarwal A., Halvorson L. M., Legradi G. (2005). Pituitary adenylate cyclase-activating polypeptide (PACAP) mimics neuroendocrine and behavioral manifestations of stress: evidence for PKA-mediated expression of the corticotropin-releasing hormone (CRH) gene. Brain Res. Mol. Brain Res. 138, 45–5710.1016/j.molbrainres.2005.03.016 - DOI - PMC - PubMed
LinkOut - more resources
Full Text Sources
Other Literature Sources