Bone regeneration in calvarial defects in a rat model by implantation of human bone marrow-derived mesenchymal stromal cell spheroids
- PMID: 26449444
- PMCID: PMC4598349
- DOI: 10.1007/s10856-015-5591-3
Bone regeneration in calvarial defects in a rat model by implantation of human bone marrow-derived mesenchymal stromal cell spheroids
Abstract
Mesenchymal stem cell (MSC) condensation contributes to membrane ossification by enhancing their osteodifferentiation. We investigated bone regeneration in rats using the human bone marrow-derived MSC-spheroids prepared by rotation culture, without synthetic or exogenous biomaterials. Bilateral calvarial defects (8 mm) were created in nude male rats; the left-sided defects were implanted with MSC-spheroids, β-tricalcium phosphate (β-TCP) granules, or β-TCP granules + MSC-spheroids, while the right-sided defects served as internal controls. Micro-computed tomography and immunohistochemical staining for osteocalcin/osteopontin indicated formation of new, full-thickness bones at the implantation sites, but not at the control sites in the MSC-spheroid group. Raman spectroscopy revealed similarity in the spectral properties of the repaired bone and native calvarial bone. Mechanical performance of the bones in the MSC-implanted group was good (50 and 60% those of native bones, respectively). All tests showed poor bone regeneration in the β-TCP and β-TCP + MSC-spheroid groups. Thus, significant bone regeneration was achieved with MSC-spheroid implantation into bone defects, justifying further investigation.
Figures
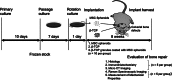
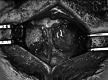
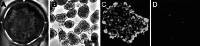
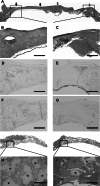
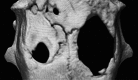
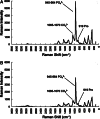
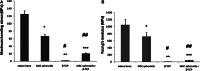
Similar articles
-
Cell-based therapy by implanted human bone marrow-derived mononuclear cells improved bone healing of large bone defects in rats.Tissue Eng Part A. 2015 May;21(9-10):1565-78. doi: 10.1089/ten.TEA.2014.0410. Epub 2015 Apr 13. Tissue Eng Part A. 2015. PMID: 25693739
-
Novel cell-free regeneration of bone using stem cell-derived growth factors.Int J Oral Maxillofac Implants. 2013 Jul-Aug;28(4):1009-16. doi: 10.11607/jomi.3036. Int J Oral Maxillofac Implants. 2013. PMID: 23869359
-
Mesenchymal stromal cells improve the osteogenic capabilities of mineralized agarose gels in a rat full-thickness cranial defect model.J Tissue Eng Regen Med. 2013 Jan;7(1):51-60. doi: 10.1002/term.495. Epub 2012 Feb 9. J Tissue Eng Regen Med. 2013. PMID: 22318970
-
Repair of canine medial orbital bone defects with miR-31-modified bone marrow mesenchymal stem cells.Invest Ophthalmol Vis Sci. 2014 Aug 28;55(9):6016-23. doi: 10.1167/iovs.14-14977. Invest Ophthalmol Vis Sci. 2014. PMID: 25168901
-
Bone Morphogenic Protein and Mesenchymal Stem Cells to Regenerate Bone in Calvarial Defects: A Systematic Review.J Clin Med. 2023 Jun 15;12(12):4064. doi: 10.3390/jcm12124064. J Clin Med. 2023. PMID: 37373757 Free PMC article. Review.
Cited by
-
Xeno-Free Spheroids of Human Gingiva-Derived Progenitor Cells for Bone Tissue Engineering.Front Bioeng Biotechnol. 2020 Aug 19;8:968. doi: 10.3389/fbioe.2020.00968. eCollection 2020. Front Bioeng Biotechnol. 2020. PMID: 32974308 Free PMC article.
-
Use of priming strategies to advance the clinical application of mesenchymal stromal/stem cell-based therapy.World J Stem Cells. 2024 Jan 26;16(1):7-18. doi: 10.4252/wjsc.v16.i1.7. World J Stem Cells. 2024. PMID: 38292438 Free PMC article.
-
Effect of Hydroxyapatite Coating by Er: YAG Pulsed Laser Deposition on the Bone Formation Efficacy by Polycaprolactone Porous Scaffold.Int J Mol Sci. 2022 Aug 12;23(16):9048. doi: 10.3390/ijms23169048. Int J Mol Sci. 2022. PMID: 36012313 Free PMC article.
-
Mesenchymal Stromal Cell Secretome: Influencing Therapeutic Potential by Cellular Pre-conditioning.Front Immunol. 2018 Dec 4;9:2837. doi: 10.3389/fimmu.2018.02837. eCollection 2018. Front Immunol. 2018. PMID: 30564236 Free PMC article. Review.
-
Enhancing survival, engraftment, and osteogenic potential of mesenchymal stem cells.World J Stem Cells. 2019 Oct 26;11(10):748-763. doi: 10.4252/wjsc.v11.i10.748. World J Stem Cells. 2019. PMID: 31692976 Free PMC article. Review.
References
-
- National Institute of Dental and Craniofacial Research (NIDCR). Prevalence (number of cases) of cleft lip and cleft palate. http://www.nidcr.nih.gov/DataStatistics/FindDataByTopic/CraniofacialBirt.... Accessed on 1 July 2012.
-
- Lane JM, Bostrom MP. Bone grafting and new composite biosynthetic graft materials. Instr Course Lect. 1998;47:525–534. - PubMed
-
- Jarcho M, Salsbury RL, Thomas MB, Doremus RH. Synthesis and fabrication of β-tricalcium phosphate (whitlockite) ceramics for potential prosthetic applications. J Mater Sci. 1979;14:142–150. doi: 10.1007/BF01028337. - DOI
Publication types
MeSH terms
LinkOut - more resources
Full Text Sources
Other Literature Sources
Research Materials