The Small Molecule Nobiletin _targets the Molecular Oscillator to Enhance Circadian Rhythms and Protect against Metabolic Syndrome
- PMID: 27076076
- PMCID: PMC4832569
- DOI: 10.1016/j.cmet.2016.03.007
The Small Molecule Nobiletin _targets the Molecular Oscillator to Enhance Circadian Rhythms and Protect against Metabolic Syndrome
Abstract
Dysregulation of circadian rhythms is associated with metabolic dysfunction, yet it is unclear whether enhancing clock function can ameliorate metabolic disorders. In an unbiased chemical screen using fibroblasts expressing PER2::Luc, we identified Nobiletin (NOB), a natural polymethoxylated flavone, as a clock amplitude-enhancing small molecule. When administered to diet-induced obese (DIO) mice, NOB strongly counteracted metabolic syndrome and augmented energy expenditure and locomotor activity in a Clock gene-dependent manner. In db/db mutant mice, the clock is also required for the mitigating effects of NOB on metabolic disorders. In DIO mouse liver, NOB enhanced clock protein levels and elicited pronounced gene expression remodeling. We identified retinoid acid receptor-related orphan receptors as direct _targets of NOB, revealing a pharmacological intervention that enhances circadian rhythms to combat metabolic disease via the circadian gene network.
Keywords: Nobiletin; circadian clock; clock amplitude-enhancing small molecule; metabolic syndrome; natural flavonoid; retinoid acid receptor-related orphan receptors (RORs).
Copyright © 2016 Elsevier Inc. All rights reserved.
Conflict of interest statement
The authors declare no conflicts of interest.
Figures
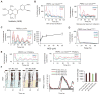
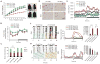
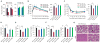
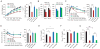
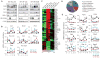
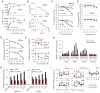
Comment in
-
_targeting Time in Metabolic Therapeutics.Cell Metab. 2016 Apr 12;23(4):575-7. doi: 10.1016/j.cmet.2016.03.011. Cell Metab. 2016. PMID: 27076073
Similar articles
-
The biological clock enhancer nobiletin ameliorates steatosis in genetically obese mice by restoring aberrant hepatic circadian rhythm.Am J Physiol Gastrointest Liver Physiol. 2022 Oct 1;323(4):G387-G400. doi: 10.1152/ajpgi.00130.2022. Epub 2022 Aug 23. Am J Physiol Gastrointest Liver Physiol. 2022. PMID: 35997288 Free PMC article.
-
Effects of the Clock Modulator Nobiletin on Circadian Rhythms and Pathophysiology in Female Mice of an Alzheimer's Disease Model.Biomolecules. 2021 Jul 9;11(7):1004. doi: 10.3390/biom11071004. Biomolecules. 2021. PMID: 34356628 Free PMC article.
-
Potent Effects of Flavonoid Nobiletin on Amplitude, Period, and Phase of the Circadian Clock Rhythm in PER2::LUCIFERASE Mouse Embryonic Fibroblasts.PLoS One. 2017 Feb 2;12(2):e0170904. doi: 10.1371/journal.pone.0170904. eCollection 2017. PLoS One. 2017. PMID: 28152057 Free PMC article.
-
The REV-ERBs and RORs: molecular links between circadian rhythms and lipid homeostasis.Future Med Chem. 2011 Apr;3(5):623-38. doi: 10.4155/fmc.11.9. Future Med Chem. 2011. PMID: 21526899 Free PMC article. Review.
-
Effect of the Citrus Flavone Nobiletin on Circadian Rhythms and Metabolic Syndrome.Molecules. 2022 Nov 10;27(22):7727. doi: 10.3390/molecules27227727. Molecules. 2022. PMID: 36431828 Free PMC article. Review.
Cited by
-
Nobiletin suppresses cholangiocarcinoma proliferation via inhibiting GSK3β.Int J Biol Sci. 2022 Sep 11;18(15):5698-5712. doi: 10.7150/ijbs.78345. eCollection 2022. Int J Biol Sci. 2022. PMID: 36263164 Free PMC article.
-
Nobiletin affects circadian rhythms and oncogenic characteristics in a cell-dependent manner.PLoS One. 2020 Jul 24;15(7):e0236315. doi: 10.1371/journal.pone.0236315. eCollection 2020. PLoS One. 2020. PMID: 32706791 Free PMC article.
-
Anti-Inflammatory Mechanisms of Dietary Flavones: Tapping into Nature to Control Chronic Inflammation in Obesity and Cancer.Int J Mol Sci. 2022 Dec 12;23(24):15753. doi: 10.3390/ijms232415753. Int J Mol Sci. 2022. PMID: 36555392 Free PMC article. Review.
-
Circadian rhythms as modulators of brain health during development and throughout aging.Front Neural Circuits. 2023 Jan 19;16:1059229. doi: 10.3389/fncir.2022.1059229. eCollection 2022. Front Neural Circuits. 2023. PMID: 36741032 Free PMC article. Review.
-
Mutations that alter Arabidopsis flavonoid metabolism affect the circadian clock.Plant J. 2022 May;110(4):932-945. doi: 10.1111/tpj.15718. Epub 2022 Mar 23. Plant J. 2022. PMID: 35218268 Free PMC article.
References
Publication types
MeSH terms
Substances
Grants and funding
- OD000895/OD/NIH HHS/United States
- R01 GM114424/GM/NIGMS NIH HHS/United States
- I01 BX000507/BX/BLRD VA/United States
- T32 AG000183/AG/NIA NIH HHS/United States
- R01AG045828/AG/NIA NIH HHS/United States
- R03 AG040583/AG/NIA NIH HHS/United States
- I01 CX000174/CX/CSRD VA/United States
- DP1 OD000895/OD/NIH HHS/United States
- R01 AG045828/AG/NIA NIH HHS/United States
- I01 BX002807/BX/BLRD VA/United States
- P30 DK056338/DK/NIDDK NIH HHS/United States
- T32AG000183/AG/NIA NIH HHS/United States
- AG040583/AG/NIA NIH HHS/United States
- P30-DK056338/DK/NIDDK NIH HHS/United States
LinkOut - more resources
Full Text Sources
Other Literature Sources
Medical
Molecular Biology Databases
Miscellaneous