Genome-scale high-resolution mapping of activating and repressive nucleotides in regulatory regions
- PMID: 27701403
- PMCID: PMC5125825
- DOI: 10.1038/nbt.3678
Genome-scale high-resolution mapping of activating and repressive nucleotides in regulatory regions
Abstract
Massively parallel reporter assays (MPRAs) enable nucleotide-resolution dissection of transcriptional regulatory regions, such as enhancers, but only few regions at a time. Here we present a combined experimental and computational approach, Systematic high-resolution activation and repression profiling with reporter tiling using MPRA (Sharpr-MPRA), that allows high-resolution analysis of thousands of regions simultaneously. Sharpr-MPRA combines dense tiling of overlapping MPRA constructs with a probabilistic graphical model to recognize functional regulatory nucleotides, and to distinguish activating and repressive nucleotides, using their inferred contribution to reporter gene expression. We used Sharpr-MPRA to test 4.6 million nucleotides spanning 15,000 putative regulatory regions tiled at 5-nucleotide resolution in two human cell types. Our results recovered known cell-type-specific regulatory motifs and evolutionarily conserved nucleotides, and distinguished known activating and repressive motifs. Our results also showed that endogenous chromatin state and DNA accessibility are both predictive of regulatory function in reporter assays, identified retroviral elements with activating roles, and uncovered 'attenuator' motifs with repressive roles in active chromatin.
Figures
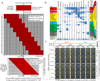
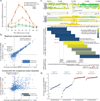
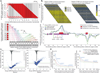
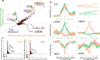
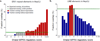
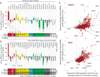
Similar articles
-
Deciphering regulatory DNA sequences and noncoding genetic variants using neural network models of massively parallel reporter assays.PLoS One. 2019 Jun 17;14(6):e0218073. doi: 10.1371/journal.pone.0218073. eCollection 2019. PLoS One. 2019. PMID: 31206543 Free PMC article.
-
High-resolution genome-wide functional dissection of transcriptional regulatory regions and nucleotides in human.Nat Commun. 2018 Dec 19;9(1):5380. doi: 10.1038/s41467-018-07746-1. Nat Commun. 2018. PMID: 30568279 Free PMC article.
-
Systematic dissection of regulatory motifs in 2000 predicted human enhancers using a massively parallel reporter assay.Genome Res. 2013 May;23(5):800-11. doi: 10.1101/gr.144899.112. Epub 2013 Mar 19. Genome Res. 2013. PMID: 23512712 Free PMC article.
-
Exploring the function of genetic variants in the non-coding genomic regions: approaches for identifying human regulatory variants affecting gene expression.Brief Bioinform. 2015 May;16(3):393-412. doi: 10.1093/bib/bbu018. Epub 2014 Jun 10. Brief Bioinform. 2015. PMID: 24916300 Review.
-
Focus on your locus with a massively parallel reporter assay.J Neurodev Disord. 2022 Sep 9;14(1):50. doi: 10.1186/s11689-022-09461-x. J Neurodev Disord. 2022. PMID: 36085003 Free PMC article. Review.
Cited by
-
Designing Cell-Type-Specific Promoter Sequences Using Conservative Model-Based Optimization.bioRxiv [Preprint]. 2024 Jun 23:2024.06.23.600232. doi: 10.1101/2024.06.23.600232. bioRxiv. 2024. PMID: 38948874 Free PMC article. Preprint.
-
Multinomial Convolutions for Joint Modeling of Regulatory Motifs and Sequence Activity Readouts.Genes (Basel). 2022 Sep 8;13(9):1614. doi: 10.3390/genes13091614. Genes (Basel). 2022. PMID: 36140783 Free PMC article.
-
Investigating enhancer evolution with massively parallel reporter assays.Genome Biol. 2018 Aug 14;19(1):114. doi: 10.1186/s13059-018-1502-5. Genome Biol. 2018. PMID: 30107810 Free PMC article.
-
Integration of high-throughput reporter assays identify a critical enhancer of the Ikzf1 gene.PLoS One. 2020 May 26;15(5):e0233191. doi: 10.1371/journal.pone.0233191. eCollection 2020. PLoS One. 2020. PMID: 32453736 Free PMC article.
-
Advances in profiling chromatin architecture shed light on the regulatory dynamics underlying brain disorders.Semin Cell Dev Biol. 2022 Jan;121:153-160. doi: 10.1016/j.semcdb.2021.08.013. Epub 2021 Sep 3. Semin Cell Dev Biol. 2022. PMID: 34483043 Free PMC article. Review.
References
MeSH terms
Substances
Grants and funding
- R01 HG008155/HG/NHGRI NIH HHS/United States
- R01 GM113708/GM/NIGMS NIH HHS/United States
- U01 HG007610/HG/NHGRI NIH HHS/United States
- U01 HG007912/HG/NHGRI NIH HHS/United States
- R01 HG006785/HG/NHGRI NIH HHS/United States
- U01 MH105578/MH/NIMH NIH HHS/United States
- U01 HG009088/HG/NHGRI NIH HHS/United States
- R01 MH109978/MH/NIMH NIH HHS/United States
- U41 HG007000/HG/NHGRI NIH HHS/United States
- RF1 AG054012/AG/NIA NIH HHS/United States
- U54 HG006991/HG/NHGRI NIH HHS/United States
- R01 HG004037/HG/NHGRI NIH HHS/United States
- R01 ES024995/ES/NIEHS NIH HHS/United States
LinkOut - more resources
Full Text Sources
Other Literature Sources
Research Materials