Generation of "Off-the-Shelf" Natural Killer Cells from Peripheral Blood Cell-Derived Induced Pluripotent Stem Cells
- PMID: 29173894
- PMCID: PMC5785702
- DOI: 10.1016/j.stemcr.2017.10.020
Generation of "Off-the-Shelf" Natural Killer Cells from Peripheral Blood Cell-Derived Induced Pluripotent Stem Cells
Abstract
Current donor cell-dependent strategies can only produce limited "made-to-order" therapeutic natural killer (NK) cells for limited patients. To provide unlimited "off-the-shelf" NK cells that serve many recipients, we designed and demonstrated a holistic manufacturing scheme to mass-produce NK cells from induced pluripotent stem cells (iPSCs). Starting with a highly accessible human cell source, peripheral blood cells (PBCs), we derived a good manufacturing practice-compatible iPSC source, PBC-derived iPSCs (PBC-iPSCs) for this purpose. Through our original protocol that excludes CD34+ cell enrichment and spin embryoid body formation, high-purity functional and expandable NK cells were generated from PBC-iPSCs. Above all, most of these NK cells expressed no killer cell immunoglobulin-like receptors (KIRs), which renders them unrestricted by recipients' human leukocyte antigen genotypes. Hence, we have established a practical "from blood cell to stem cells and back with less (less KIRs)" strategy to generate abundant "universal" NK cells from PBC-iPSCs for a wide range of patients.
Keywords: cancer; cell therapy; cytotoxicity; immunotherapy; induced pluripotent stem cells; killer cell immunoglobulin-like receptors; natural killer cells; peripheral blood cells.
Copyright © 2017 The Authors. Published by Elsevier Inc. All rights reserved.
Figures
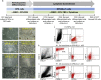
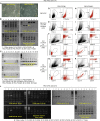
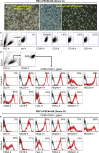
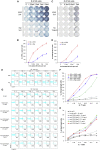
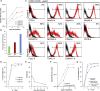
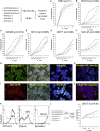
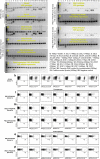
Similar articles
-
Clinical-scale derivation of natural killer cells from human pluripotent stem cells for cancer therapy.Stem Cells Transl Med. 2013 Apr;2(4):274-83. doi: 10.5966/sctm.2012-0084. Epub 2013 Mar 20. Stem Cells Transl Med. 2013. PMID: 23515118 Free PMC article.
-
Generation of universal natural killer cells from a cryopreserved cord blood mononuclear cell-derived induced pluripotent stem cell library.FEBS Open Bio. 2022 Oct;12(10):1771-1781. doi: 10.1002/2211-5463.13460. Epub 2022 Jul 1. FEBS Open Bio. 2022. PMID: 35747945 Free PMC article.
-
An Improved Method to Produce Clinical-Scale Natural Killer Cells from Human Pluripotent Stem Cells.Methods Mol Biol. 2019;2048:107-119. doi: 10.1007/978-1-4939-9728-2_12. Methods Mol Biol. 2019. PMID: 31396935
-
Off-the-shelf cell therapy with induced pluripotent stem cell-derived natural killer cells.Semin Immunopathol. 2019 Jan;41(1):59-68. doi: 10.1007/s00281-018-0721-x. Epub 2018 Oct 25. Semin Immunopathol. 2019. PMID: 30361801 Review.
-
iPSC-Derived Natural Killer Cells for Cancer Immunotherapy.Mol Cells. 2021 Aug 31;44(8):541-548. doi: 10.14348/molcells.2021.0078. Mol Cells. 2021. PMID: 34373366 Free PMC article. Review.
Cited by
-
Adoptive Immunotherapy: A Human Pluripotent Stem Cell Perspective.Cells Tissues Organs. 2023;212(5):439-467. doi: 10.1159/000528838. Epub 2023 Jan 4. Cells Tissues Organs. 2023. PMID: 36599319 Free PMC article. Review.
-
Technical advances in NK cell-based cellular immunotherapy.Cancer Biol Med. 2019 Nov;16(4):647-654. doi: 10.20892/j.issn.2095-3941.2019.0187. Cancer Biol Med. 2019. PMID: 31908885 Free PMC article.
-
NK Cell Plasticity in Cancer.J Clin Med. 2019 Sep 19;8(9):1492. doi: 10.3390/jcm8091492. J Clin Med. 2019. PMID: 31546818 Free PMC article. Review.
-
Recent Updates on Induced Pluripotent Stem Cells in Hematological Disorders.Stem Cells Int. 2019 May 2;2019:5171032. doi: 10.1155/2019/5171032. eCollection 2019. Stem Cells Int. 2019. PMID: 31191673 Free PMC article. Review.
-
Induced pluripotent stem cell-based disease modeling and prospective immune therapy for coronavirus disease 2019.Cytotherapy. 2022 Mar;24(3):235-248. doi: 10.1016/j.jcyt.2021.08.003. Epub 2021 Sep 14. Cytotherapy. 2022. PMID: 34656419 Free PMC article. Review.
References
-
- Benson D.M., Jr., Cohen A.D., Jagannath S., Munshi N.C., Spitzer G., Hofmeister C.C., Efebera Y.A., Andre P., Zerbib R., Caligiuri M.A. A phase I trial of the anti-KIR antibody IPH2101 and lenalidomide in patients with relapsed/refractory multiple myeloma. Clin. Cancer Res. 2015;21:4055–4061. - PMC - PubMed
-
- Colucci F., Caligiuri M.A., Di Santo J.P. What does it take to make a natural killer? Nat. Rev. Immunol. 2003;3:413–425. - PubMed
-
- De Obaldia M.E., Bhandoola A. Transcriptional regulation of innate and adaptive lymphocyte lineages. Annu. Rev. Immunol. 2015;33:607–642. - PubMed
Publication types
MeSH terms
Substances
LinkOut - more resources
Full Text Sources
Other Literature Sources
Research Materials