Functional principles of steerable multi-element probes in insects
- PMID: 30259619
- PMCID: PMC7379267
- DOI: 10.1111/brv.12467
Functional principles of steerable multi-element probes in insects
Abstract
Hemipterans, mosquitoes, and parasitic wasps probe in a variety of substrates to find hosts for their larvae or food sources. Probes capable of sensing and precise steering enable insects to navigate through solid substrates without visual information and to reach _targets that are hidden deep inside the substrate. The probes belong to non-related taxa and originate from abdominal structures (wasps) or mouthparts (hemipterans and mosquitoes), but nevertheless share several morphological characteristics. Although the transport function clearly differs (egg laying and acquisition of liquid food), the functional demands on the mechanical behaviour of the probe within the substrate tend to be similar. The probe needs to be thin to limit substrate deformation, and long, in order to attain substantial path lengths or depths. We linked the morphology across taxa to the different functional requirements, to provide insights into the biology of probing insects and the evolution of their probes. Current knowledge of insect probes is spread over many taxa, which offers the possibility to derive general characteristics of insect probing. Buckling during initial puncturing is limited by external support mechanisms. The probe itself consist of multiple (3-6) parts capable of sliding along one another. This multi-part construction presumably enables advancement and precise three-dimensional steering of the probe through the substrate with very low net external pushing forces, preventing buckling during substrate penetration. From a mechanical viewpoint, a minimum of three elements is required for 3D steering and volumetric exploration, as realised in the ovipositors of wasps. More elements, such as in six-element probes of mosquitoes, may enhance friction in soft substrates. Alternatively, additional elements can have functions other than 'drilling', such as saliva injection in mosquitoes. Despite the gross similarities, probes show differences in their cross sections, tip morphologies, relative lengths of their elements, and the shape of their interconnections. The hypothesis is that the probe morphology is influenced by the substrate properties, which are mostly unknown. Correlating the observed diversity to substrate-specific functional demands is therefore currently impossible. We conclude that a multipart probe with sliding elements is highly effective for volumetric substrate probing. Shared functional demands have led to an evolutionary convergence of slender multi-element probes in disparate insect taxa. To fully understand 3D probing, it is necessary to study the sensory and material properties, as well as the detailed kinematics and dynamics of the various probes in relation to the nature of the selective pressure originating from the species-specific substrates. Such knowledge will deepen our understanding of probing mechanisms and may support the development of slender, bio-inspired probes.
Keywords: buckling avoidance; hemipterans; mosquitoes; multi-element probes; parasitic wasps; spatial probing; steering.
© 2018 The Authors. Biological Reviews published by John Wiley & Sons Ltd on behalf of Cambridge Philosophical Society.
Figures
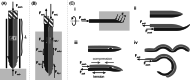
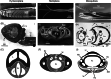
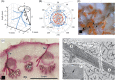
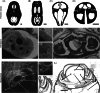
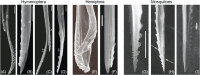
Similar articles
-
Mechanisms of ovipositor insertion and steering of a parasitic wasp.Proc Natl Acad Sci U S A. 2017 Sep 12;114(37):E7822-E7831. doi: 10.1073/pnas.1706162114. Epub 2017 Aug 28. Proc Natl Acad Sci U S A. 2017. PMID: 28847936 Free PMC article.
-
Stiffness gradients facilitate ovipositor bending and spatial probing control in a parasitic wasp.J Exp Biol. 2019 May 2;222(Pt 9):jeb195628. doi: 10.1242/jeb.195628. J Exp Biol. 2019. PMID: 30952686
-
The ovipositor actuation mechanism of a parasitic wasp and its functional implications.J Anat. 2020 Oct;237(4):689-703. doi: 10.1111/joa.13216. Epub 2020 Jun 13. J Anat. 2020. PMID: 32533567 Free PMC article.
-
Making the cut: mechanics of cutting and steering of insect probes.Curr Opin Insect Sci. 2020 Dec;42:84-89. doi: 10.1016/j.cois.2020.09.013. Epub 2020 Oct 7. Curr Opin Insect Sci. 2020. PMID: 33038534 Review.
-
Insect societies and the social brain.Curr Opin Insect Sci. 2016 Jun;15:1-8. doi: 10.1016/j.cois.2016.01.010. Epub 2016 Feb 4. Curr Opin Insect Sci. 2016. PMID: 27436726 Review.
Cited by
-
Biologically Inspired Surgical Needle Steering: Technology and Application of the Programmable Bevel-Tip Needle.Biomimetics (Basel). 2020 Dec 16;5(4):68. doi: 10.3390/biomimetics5040068. Biomimetics (Basel). 2020. PMID: 33339448 Free PMC article.
-
Obstacle negotiation in female desert locust oviposition digging.Naturwissenschaften. 2024 Aug 8;111(5):43. doi: 10.1007/s00114-024-01929-1. Naturwissenschaften. 2024. PMID: 39115554 Free PMC article.
-
Terebra steering in chalcidoid wasps.Front Zool. 2023 Aug 8;20(1):26. doi: 10.1186/s12983-023-00503-1. Front Zool. 2023. PMID: 37553687 Free PMC article.
-
Biomimetics with Trade-Offs.Biomimetics (Basel). 2023 Jun 17;8(2):265. doi: 10.3390/biomimetics8020265. Biomimetics (Basel). 2023. PMID: 37366860 Free PMC article.
-
Comparative Material and Mechanical Properties among Cicada Mouthparts: Cuticle Enhanced with Inorganic Elements Facilitates Piercing through Woody Stems for Feeding.Biology (Basel). 2023 Jan 29;12(2):207. doi: 10.3390/biology12020207. Biology (Basel). 2023. PMID: 36829484 Free PMC article.
References
-
- Ahmad, A. , Kaushik, S. , Ramamurthy, V. V. , Lakhanpaul, S. , Ramani, R. , Sharma, K. K. & Vidyarthi, A. S. (2012). Mouthparts and stylet penetration of the lac insect Kerria lacca (Kerr) (Hemiptera:Tachardiidae). Arthropod Structure & Development 41, 435–441. - PubMed
-
- Ahmed, T. , Zhang, T. , He, K. , Bai, S. & Wang, Z. (2013). Sense organs on the ovipositor of Macrocentrus cingulum Brischke (Hymenoptera: Braconidae): their probable role in stinging, oviposition and host selection process. Journal of Asia‐Pacific Entomology 16, 343–348.
-
- Alves, T. J. S. , Wanderly‐Teixeira, V. , Teixeria, A. A. C. , Silva‐Torres, C. S. A. , Malaquias, J. B. , Pereira, B. F. , Caetano, F. H. & Cunha, F. M. (2014). Parasitoid‐host interaction: sensory structures involved in the parasitism behavior of Bracon vulgaris (Hymenoptera: Braconidae). Animal Biology 64, 365–381.
-
- Anderson, W. G. , Heng‐Moss, T. M. , Baxendale, F. P. , Baird, L. M. , Sarath, G. & Higley, L. (2006). Chinch bug (Hemiptera: Blissidae) mouthpart morphology, probing frequencies, and locations on resistant and susceptible germplasm. Journal of Economic Entomology 99, 212–221. - PubMed
-
- Angelini, D. R. & Kaufman, T. C. (2004). Functional analyses in the hemipteran Oncopeltus fasciatus reveal conserved and derived aspects of appendage patterning in insects. Developmental Biology 271, 306–321. - PubMed
Publication types
MeSH terms
LinkOut - more resources
Full Text Sources
Other Literature Sources