An Evaluation of the In Vitro Roles and Mechanisms of Silibinin in Reducing Pyrazinamide- and Isoniazid-Induced Hepatocellular Damage
- PMID: 32466226
- PMCID: PMC7279482
- DOI: 10.3390/ijms21103714
An Evaluation of the In Vitro Roles and Mechanisms of Silibinin in Reducing Pyrazinamide- and Isoniazid-Induced Hepatocellular Damage
Abstract
Tuberculosis remains a significant infectious lung disease that affects millions of patients worldwide. Despite numerous existing drug regimens for tuberculosis, drug-induced liver injury is a major challenge that limits the effectiveness of these therapeutics. Two drugs that form the backbone of the commonly administered quadruple antitubercular regimen, that is, pyrazinamide (PZA) and isoniazid (INH), are associated with such hepatotoxicity. Yet, we lack safe and effective alternatives to the antitubercular regimen. Consequently, current research largely focuses on exploiting the hepatoprotective effect of nutraceutical compounds as complementary therapy. Silibinin, a herbal product widely believed to protect against various liver diseases, potentially provides a useful solution given its hepatoprotective mechanisms. In our study, we identified silibinin's role in mitigating PZA- and INH-induced hepatotoxicity and elucidated a deeper mechanistic understanding of silibinin's hepatoprotective ability. Silibinin preserved the viability of human foetal hepatocyte line LO2 when co-administered with 80 mM INH and decreased apoptosis induced by a combination of 40 mM INH and 10 mM PZA by reducing oxidative damage to mitochondria, proteins, and lipids. Taken together, this proof-of-concept forms the rational basis for the further investigation of silibinin's hepatoprotective effect in subsequent preclinical studies and clinical trials.
Keywords: drug-induced liver injury (DILI); isoniazid; oxidative stress; pyrazinamide; silibinin; tuberculosis.
Conflict of interest statement
The authors report no conflict of interests or competing financial interests.
Figures
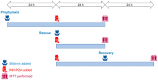
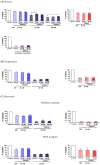
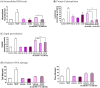
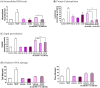
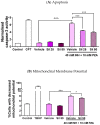
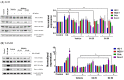
Similar articles
-
Protective effect of a 50% hydroalcoholic fruit extract of Emblica officinalis against anti-tuberculosis drugs induced liver toxicity.Phytother Res. 2005 Mar;19(3):193-7. doi: 10.1002/ptr.1631. Phytother Res. 2005. PMID: 15934014
-
Terminalia chebula (fruit) prevents liver toxicity caused by sub-chronic administration of rifampicin, isoniazid and pyrazinamide in combination.Hum Exp Toxicol. 2006 Mar;25(3):111-8. doi: 10.1191/0960327106ht601oa. Hum Exp Toxicol. 2006. PMID: 16634329
-
Isoniazid and its toxic metabolite hydrazine induce in vitro pyrazinamide toxicity.Int J Antimicrob Agents. 2008 Jun;31(6):577-80. doi: 10.1016/j.ijantimicag.2008.01.022. Epub 2008 Mar 21. Int J Antimicrob Agents. 2008. PMID: 18358703
-
Hepatotoxicity mechanisms of isoniazid: A mini-review.J Appl Toxicol. 2015 Dec;35(12):1427-32. doi: 10.1002/jat.3175. Epub 2015 Jun 10. J Appl Toxicol. 2015. PMID: 26095833 Review.
-
Metabolism and Hepatotoxicity of Pyrazinamide, an Antituberculosis Drug.Drug Metab Dispos. 2021 Aug;49(8):679-682. doi: 10.1124/dmd.121.000389. Epub 2021 Jun 1. Drug Metab Dispos. 2021. PMID: 34074731 Free PMC article. Review.
Cited by
-
The Potential Application of Chinese Medicine in Liver Diseases: A New Opportunity.Front Pharmacol. 2021 Nov 4;12:771459. doi: 10.3389/fphar.2021.771459. eCollection 2021. Front Pharmacol. 2021. PMID: 34803712 Free PMC article. Review.
-
Herbal and dietary supplement induced liver injury: Highlights from the recent literature.World J Hepatol. 2021 Sep 27;13(9):1019-1041. doi: 10.4254/wjh.v13.i9.1019. World J Hepatol. 2021. PMID: 34630872 Free PMC article. Review.
-
Nutritional intervention is promising in alleviating liver injury during tuberculosis treatment: a review.Front Nutr. 2023 Sep 21;10:1261148. doi: 10.3389/fnut.2023.1261148. eCollection 2023. Front Nutr. 2023. PMID: 37810929 Free PMC article. Review.
-
Herbo-vitamin medicine Livogrit Vital ameliorates isoniazid induced liver injury (IILI) in human liver (HepG2) cells by decreasing isoniazid accumulation and oxidative stress driven hepatotoxicity.BMC Complement Med Ther. 2024 Nov 8;24(1):386. doi: 10.1186/s12906-024-04685-x. BMC Complement Med Ther. 2024. PMID: 39516811 Free PMC article.
-
Hepatoprotective activity of melittin on isoniazid- and rifampicin-induced liver injuries in male albino rats.BMC Pharmacol Toxicol. 2021 Jul 3;22(1):39. doi: 10.1186/s40360-021-00507-9. BMC Pharmacol Toxicol. 2021. PMID: 34217369 Free PMC article.
References
-
- Global Tuberculosis Report 2019. World Health Organization; Geneva, Switzerland: 2019.
-
- Abbara A., Chitty S., Roe J.K., Ghani R., Collin S.M., Ritchie A., Kon O.M., Dzvova J., Davidson H., Edwards T.E., et al. Drug-induced liver injury from antituberculous treatment: A retrospective study from a large TB centre in the UK. BMC Infect. Dis. 2017;17:231. doi: 10.1186/s12879-017-2330-z. - DOI - PMC - PubMed
MeSH terms
Substances
Grants and funding
LinkOut - more resources
Full Text Sources
Medical