05 January 2025: Clinical Research
Comparative Analysis of Gelatin/Polylactic Acid and Commercial PLA Membranes for Guided Bone Regeneration: A Randomized Clinical Trial
Jing Wang1ABCDEFG, Wentian Chen2BCD, Min Huang3ACDF, Zhitong Zhong4BCF, Pei Wang5ACDEF, Runfa Wu3ABCDEFG*DOI: 10.12659/MSM.944713
Med Sci Monit 2025; 31:e944713
Abstract
BACKGROUND: This study included 32 patients with single missing teeth and alveolar bone defects and aimed to compare outcomes from guided bone regeneration with a gelatin/polylactic acid (GT/PLA) barrier membrane and a Guidor® bioresorbable matrix barrier dental membrane.
MATERIAL AND METHODS: A total of 32 participants were recruited in the clinical study, with single missing teeth and alveolar bone defects, requiring guided bone regeneration (32 missing teeth in total). They were randomly divided into the GT/PLA membrane group (experimental) and Guidor® membrane group (control) by the envelope method (n=16). Both membranes were used intraoperatively to cover the bone substitute material. Cone beam computed tomography (CBCT) was performed immediately and at 6 months after surgery to assess the amount of bone resorption. In addition, the osteogenic efficacy was calculated. The soft tissue index (STI), wound healing, membrane exposure, and incidence of infection in the operative area were evaluated.
RESULTS: The implant survival rate was 100% in both groups. The average bone resorption was 148.54±107.42 mm³ in the experimental group and 185.25±85.31 mm³ in the control group (P=0.163); the osteogenic efficacy was 75% in the experimental group and 56% in the control group (P=0.458). Moreover, the parameters of STI, wound healing, membrane exposure, and incidence of infection in the operative area showed no statistically significant difference between the 2 groups (P>0.05).
CONCLUSIONS: The GT/PLA barrier membrane yielded non-inferior clinical and imaging results to the GUIDOR® membrane, exhibiting good efficacy and biocompatibility in GBR.
Keywords: Dental Implants, Gelatin, Poly(lactide)
Introduction
Alveolar bone loss due to periodontitis, dental caries, and trauma poses a challenge for dentists in dental restoration. Current treatments regarding alveolar bone regeneration for better survival of natural teeth or dental implants include sinus lift, socket lift, guided bone regeneration (GBR), and socket preservation [1]. These methods can be used to augment the missing alveolar bone, so that the tooth or implant is surrounded by enough bone tissue to become established. Over the past 30 years, many studies have demonstrated that GBR is a successful and reliable bone augmentation technique [2–5]. It has been widely applied to supplement peri-implant bone and in many patients with missing teeth. The principle of GBR is to create a barrier between the soft tissue and the bone defect through the barrier membranes, selectively blocking epithelial cells and fibroblasts from the defect so that the bone tissue can be repaired and regenerated without interference [6]. GBR follows the “PASS” principle – Primary wound closure, Angiogenesis, Space maintenance, and Stability. Therefore, creating a stable osteogenic space is essential for GBR, with the barrier membrane playing an essential role [7,8]. A systematic meta-analysis concluded that the mean marginal bone level (MBL) was significantly higher in bone grafts with barrier membrane coverage compared to the non-covered group [9].
Clinical GBR barrier membranes are mainly categorized into non-absorbable and absorbable membranes [10–14]. Currently, among all the absorbable barrier membranes, collagen-based absorbable membranes are the first choice for oral implantation [15]. Clinical feedback shows that this type of material effectively fulfills its barrier role in oral bone augmentation procedures [16,17]. However, the material has poor mechanical strength and low tensile strength, and easily tears and fractures during surgery. In such cases, the collapse of the material compromises its ability to maintain space during the application process, negatively impacting the final restoration effect [18]. Furthermore, collagen products have a fast degradation rate, with an excessively short barrier effect time [19]. To overcome these drawbacks, researchers have found that collagen membranes treated with cross-linking techniques can improve the mechanical properties of collagen membranes. Commonly used cross-linking methods include physical methods such as UV cross-linking, thermal cross-linking, and chemical reagents such as glutaraldehyde (GLUT) and epigallocatechin gallate (EGCG). Gayathri et al found that the fibronectin network of GLUT cross-linked collagen membranes was denser, which could improve their mechanical properties [20]. Another study found that EGCG cross-linked collagen membranes not only down-regulated the expression of inflammatory factors, but also recruited CD206+ M2 macrophages and promoted the expression of growth factors [21]. However, highly cross-linked membranes have a higher likelihood of postoperative exposure after surgery, and the cytotoxicity of residual cross-linking agents and altered membrane permeability after cross-linking may contribute to poor GBR treatment outcomes [22].
With the development of medical polymers in recent years, polymer membranes made from synthetic materials have emerged [23–26]. Among them, polylactic acid (PLA), a biomaterial certified and approved for use in the human body by the US Food and Drug Administration (FDA), has shown promising applications owing to its good surface hydrophilicity, biocompatibility, and biosafety properties [26–29]. In addition, it is one of the most widely researched biodegradable polymers in tissue engineering. However, PLA polymers produce acidic products during the degradation process, which decreases the local pH value and causes oral inflammation. In addition, due to the technical limitations in processing and preparation, most of the oral restorative membranes products on the market consist of double-layer structures. This results in a thicker product, which produces an occupancy effect, thereby affecting the suture closure; the tension produced by the gums can also result in the deformation of the bone powder or resorption, leading to failure of oral bone regeneration [30].
As a biomedical material, gelatin has good biocompatibility and biodegradability, and the molecule contains many RGD (Arg-Gly-Asp) peptides, which are favorable for cell adhesion and growth [31,32]. Regarding the acid production by PLA degradation, gelatin has the advantage that it can be degraded into amino acids and peptides in vivo and decomposed into α-keto acids, amines, and carbon dioxide through deamination, transamination, combined deamination, or decarboxylation. The α-keto acids generated from amino acid catabolism can be converted into sugars or lipids, re-synthesized into certain non-essential amino acids, or oxidized to carbon dioxide and water through the tricarboxylic acid cycle, thereby causing less local inflammation [33]. In addition, gelatin is widely available and inexpensive, providing an economic incentive for enhancing its practical application [34]. Combining the advantages of both materials, gelatin/polylactic acid (GT/PLA) can act as a guided bone regeneration membrane; it can be a good barrier to external soft tissue cells and also provides good bioactivity. [35].
Currently, there are relatively few types of clinically degradable synthetic polymer membranes. Several manufacturers introduced such products about 15 years ago, but due to the backwardness of the manufacturing process at that time, the performance of polymer membranes could not fully meet the clinical needs; therefore, they were gradually replaced by collagen membranes. With the development of manufacturing processes, polymers have become easier to handle and mold. For further development, we will evaluate a gelatin/polylactic acid (GT/PLA) barrier membrane using the additive manufacturing process. The membrane has a bionic 3-dimensional network structure with good strength, toughness, handling, softness, permeability, and barrier effect (patent-protected). It does not affect the exchange of small molecules between tissues in the defect area. The GT/PLA membrane showed good mechanical properties and biocompatibility in the pilot experiment [36].
To determine the biocompatibility and efficacy of GT/PLA membrane in guided bone regeneration techniques, we designed a randomized, single-blind, positively controlled, non-inferiority study with Guidor® PLA membranes. Guidor® (Paramus, NJ, USA) is a commercial synthetic membrane that contains macropores for bone and vascular regeneration and is made from a biphasic calcium phosphate compound consisting of hydroxyapatite and β-tricalcium phosphate (β-TCP). This clinical exploratory study evaluated the primary effectiveness index of effective bone turnover rate, as well as secondary effectiveness indexes such as soft tissue index, wound healing, and membrane exposure and infection in the operative area, demonstrating the clinical value and social significance of polymeric barrier membranes.
The present study included 32 patients with single missing teeth and alveolar bone defects and compared outcomes from guided bone regeneration with a gelatin/polylactic acid (GT/PLA) barrier membrane and a Guidor® bioresorbable matrix barrier dental membrane.
Material and Methods
STUDY DESIGN:
Sample size estimation was performed using G*Power software 3.1.9 (Heinrich Heine University Dusseldorf, Dusseldorf, Germany). When the study power was set to 0.8, the α error to 0.05, and the effect size to 0.5, the total sample size was estimated to be 32. A total of 36 subjects were screened to ensure a sufficient total sample size, out of which 4 subjects withdrew from the study midway due to personal reasons, thus resulting in the final inclusion of 32 subjects (16 in each group) (Figure 1).
The clinical study subjects were patients with oral alveolar bone dehiscence defects requiring guided bone regeneration (GBR). A total of 36 patients were screened and 32 subjects were finally included, while 4 patients were excluded. All patients were randomly assigned by envelope lottery at a 1: 1 ratio to the experimental group or the control group. The experimental group patients were treated with the experimental product as GT/PLA barrier membranes (Medprin Regenerative Medical Technologies Co., Ltd, Guangdong, China), length×width: 15×20 mm, 20×30 mm, 30×40 mm, the experimental membranes do not need to distinguish between positive and negative sides and is easy to operate. Control group patients were treated with the control products, a commercially Guidor® resorbable PLA membranes (Sunstar Americas Inc, Chicago, America), length×width: 15×20 mm, 20×28 mm. The primary effectiveness index for clinical evaluation was osteogenic efficacy, and the secondary effectiveness indexes included soft tissue index (STI) and wound healing. Moreover, the safety indexes included membrane exposure and incidence of infection in the operative area. All clinicians involved in the study were trained and qualified in the new version of the Good Clinical Practice (GCP) for drug clinical studies, and the surgical and data measurement processes were homogeneous.
INCLUSION CRITERIA:
(1) No sex restriction, patients aged 18–65 years old; (2) Scheduled implant restoration due to single missing teeth, with the presence of neighboring teeth on at least 1 side, and CBCT (Beijing Langshi Equipment Co., Ltd., Beijing, China) imaging assessment of horizontal bone defects requiring GBR; (3) Patients and/or guardians agreed to participate in this study and signed an informed consent form.
EXCLUSION CRITERIA:
(1) Preservation of the remaining 4 bone walls at the extraction socket sites; (2) Use of metal porcelain crowns or metal casting pins in the neighboring teeth; (3) Presence of acute or chronic infection in the operation area; (4) Participation in a similar study or other interventional clinical studies within 30 days before signing the informed consent form; (5) History of allergic reaction to gelatin, a history of severe allergic diseases, or a history of severe immunodeficiencies; (6) Uncontrolled metabolic diseases, such as type 1 diabetes mellitus, severe hepatic and renal dysfunction; (7) Long-term use of steroids; (8) History of drug addiction, alcoholism, or smoking more than 10 cigarettes/day for 6 months prior to participation in this study; (9) Pregnant and lactating women; (10) Poor compliance; (11) Other conditions that in the judgment of the investigator make participation in the study unsuitable.
RANDOMIZATION:
Random grouping was performed by envelope drawing method. Random number tables for instrument randomization were provided by statistical professionals, and the preparation of the randomization envelopes was completed by someone other than the investigator of this study. After obtaining informed consent from the subject and determining that the inclusion exclusion criteria were met, the researcher opened the envelopes sequentially, identified the group of participants, and performed the corresponding surgical procedure.
BLINDING METHOD:
The subject and observer in this study were both blinded. Due to the different appearance of the experimental and control products, it is not possible to blind the operator. Therefore, the surgeon did not perform follow-up observational assessments, and similarly, the observer did not participate in the surgical treatment and clinical operations.
SURGICAL TREATMENT:
The steps of surgical treatment were the same in the experimental and control groups. A single dose of antibiotics (amoxicillin dispersible tablets, tinidazole tablets) and fenbendazole extended-release capsules were given as prophylaxis 0.5–2 h before surgery. Chlorhexidine was gargled for 1 minute in 3 divided doses, and the maxillofacial skin and oral cavity were routinely disinfected with iodophor solution containing 0.5% effective iodine. A local injection of 2% Scandonest (Septodont, Paris, France) epinephrine was used for infiltration anesthesia. A transverse incision was made at the top of the alveolar ridge, and an intragingival crevicular incision was made on the side of the adjacent gap. The full-thickness flap was turned, the hole was prepared step by step, and the implant (Straumann, Switzerland; Dentium, Korea) was inserted. Trophic holes were prepared lateral to the buccal bone defects. Natural bone mineral of bovine origin (Bio-Oss Spongiosa Granules, particle size 0.25–1 mm; Geistlich Pharma AG, Wolhusen, Switzerland) was used as the bone grafting material for all patients in this study. Intraoperatively, either an experimental group membrane or a control group membrane was used, depending on the patient’s preoperative envelope draw. The barrier membrane was placed over the defect and the edge of the membrane was tightened against the alveolar bone. If necessary, the membranes were fixed using membrane staples to avoid membrane sliding. Finally, a reduction suture was carried out to close the wound completely (Figure 2). Submerged healing was used in all cases. CBCT was performed on the same day after surgery.
CLINICAL EVALUATION INDEXES AND PARAMETERS:
In the clinical study, the primary effectiveness index was the osteogenic efficacy, and the secondary effectiveness indexes were soft tissue index (STI) and wound healing. Safety was determined by the incidence of membrane exposure and the incidence of infection in the operation area. All test data measurements and verifications were conducted by a third-party measurement team composed of experts with relevant professional backgrounds who were not involved in the clinical study. The measurement team was blinded during the data flow process.
PRIMARY EFFECTIVENESS INDICATOR – EFFECTIVE BONE TURNOVER RATE:
Each patient underwent CBCT within 30 days before the operation, on the postoperative day, and 6 months postoperatively (Figure 3). Bone volume measurement was performed by a third party through imaging results. The preoperative, immediate postoperative, and 6-month postoperative CBCT data were exported to Digital Imaging and Communications in Medicine (DICOM) format and imported into Mimics 21.0 (Materialize, Leuven, Belgium) software. A new bone graft model was established immediately after surgery and an osteogenic model was established 6 months after surgery, and Boolean operations were used to obtain the volume of bone grafted after surgery and the volume of bone formed 6 months later, and the difference between the 2 was the bone resorption volume, which was then used to calculate the bone resorption rate. Bone resorption rate={(bone volume on the day of surgery - bone volume before surgery) - (bone volume 6 months after surgery - bone volume before surgery)}/(bone volume on the day of surgery - bone volume before surgery) ×100%. The effective bone turnover was defined as a bone resorption rate <50%; ineffective bone turnover was defined as a bone resorption rate ≥50%. The effective bone turnover rate was calculated based on the effective cases. The management and evaluation process of CBCT data is detailed in Standard Operating Procedures for CBCT Data Management and Evaluation.
SECONDARY EFFECTIVENESS INDICATORS:
The soft tissue index and wound healing were assessed 2 weeks postoperatively.
SOFT TISSUE INDEX (STI):
Score 0: the soft tissues in the area covered by the oral membrane were not inflamed and had a normal appearance; Score 1: no inflammation in most of the soft tissue of the area covered by the oral membrane, with slight inflammation in the gingival margins; Score 2: redness in the soft tissue of the area covered by the oral membrane, without swelling or suppuration; Score 3: redness in the soft tissue of the area covered by the oral membrane, with swelling or suppuration [37].
WOUND HEALING:
Grade A: excellent healing, initial healing with no adverse reaction; Grade B: poor healing with inflammatory reaction at the healing site, such as redness, swelling, hardness, hematoma, and subcutaneous effusion, but not suppurative; Grade C: poor healing, suppurative, and requiring incision and drainage.
CLINICAL SAFETY INDICATORS:
The patients were monitored for membrane exposure and infection in the operative area at 2 weeks postoperatively and 6 months postoperatively. The incidence of all adverse events during the study period was recorded.
DATA ANALYSIS:
Descriptive statistics are expressed as mean, frequency, and standard deviation. Clinical and imaging results were tested for normality and homogeneity of variance using the Shapiro-Wilk method and Levene’s test, respectively. For comparisons between the 2 groups, an independent samples
Results
SAMPLE CHARACTERISTICS:
A total of 36 patients were screened in this study, and after excluding 4 cases according to the exclusion criteria, 32 subjects were finally included. All the patients were randomly assigned to the experimental or control group by drawing envelopes provided by third-party staff within 1 hour before surgery. The experimental group consisted of 16 patients, including 4 males and 12 females, with a mean age of 43.44 ± 11.37 years (range 20~58 years). The control group had 16 patients, including 5 males and 11 females, with a mean age of 48.13±11.87 years (range 22~64 years) (P=0.263). During the 6-month follow-up period, no implant detachment was found in either group, showing an implant survival rate of 100% (P>0.05). No statistically significant differences in basic characteristics were observed between the 2 groups, including sex and age differences and implant survival rates (Table 1).
EFFECTIVE BONE TURNOVER RATE:
The CBCT imaging results on the day after the operation revealed an average bone graft volume of 350.35±119.86 mm3 in the experimental group and 390.99±147.74 mm3 in the control group, demonstrating no statistically significant difference between the 2 groups (P=0.451). After 6 months of hard tissue healing, the average bone formation was 201.80±117.69 mm3 in the experimental group and 205.74±134.76 mm3 in the control group, showing no statistically significant difference between the 2 groups (P=0.913). In addition, the average bone resorption in the experimental group was 148.54±107.42 mm3 and the average bone resorption in the control group was 185.25±85.31 mm3, revealing no statistically significant difference between the test group and the control group (P=0.163). The bone resorption rate was (42.9±24)% in the experimental group and (49.3±23)% in the control group (P=0.453). Furthermore, the effective number of osteogenic cases was 12 in the experimental group and 9 in the control group, so the osteogenic efficacy was higher in the experimental group than in the control group (75% vs 56%), although there was no statistically significant difference in osteogenic efficacy between the 2 groups (P=0.458) (Table 2).
SOFT TISSUE INDEX AND WOUND HEALING:
At 2 weeks postoperatively, both groups showed good soft tissue healing. In the experimental group, 11 patients scored 0 points in the soft tissue index, 4 cases scored 1 point, and 1 case scored 2 points. In the control group, 12 cases scored 0 points in the soft tissue index, 3 cases scored 1 point, and 1 case scored 2 points. No statistically significant difference was observed in the soft tissue index between the 2 groups (P=0.719) (Table 3). In addition, the experimental group had 14 patients with grade A healing and 2 patients with grade B healing, the same as in the control group, and there were no patients with grade C healing at the 2-week follow-up.
MEMBRANE EXPOSURE AND INFECTION:
At 2–3 months postoperatively, 1 patient in each group developed localized redness, swelling, and inflammation in the operated area, which resolved over time with anti-inflammatory treatment, and the final restoration was completed at 6 months with good osteogenesis and a stable implant. No membrane exposure occurred during this period. Therefore, no statistically significant difference in the secondary effectiveness indicators was found between the 2 groups.
Discussion
In this study, the experimental and control groups both had a 100% implant survival rate during the 6-month follow-up period. In clinical practice, the short-term effect of GBR is usually judged by CBCT photography combined with clinical observation at 6 months postoperatively. The results of CBCT 3D reconstruction showed that both groups exhibited some bone resorption, and the amount of bone resorption in the experimental group was less compared with the control group, which means that the osteogenic efficacy was higher, at about 75%, although the results of the 2 groups were not significantly different, and the osteogenic efficacy was higher than 50% in both groups. The soft tissue healing index was good in both groups. This demonstrated the good osteogenic effectiveness of PLA in GBR.
The natural periosteum plays an essential role in GBR by storing progenitor cells, as well as serving as a source of local growth factors and a scaffold for recruiting cells and growth factors [38]. However, natural periosteum is a limited source, and it is immunogenic and expensive. Therefore, as a direct replacement for natural periosteum, tissue regeneration-engineered periosteum is the synthesis of artificial periosteum using biomimetic methods to produce membranous tissue with similar function and structure to natural periosteum. Numerous tests have shown that polymeric polymers can have good mechanical strength to maintain the space for new bone, which can be controlled with appropriate pore size for the attachment of osteoblasts [39]. Excellent porosity facilitates cell proliferation and nutrient availability while inhibiting fibroblast infiltration from soft tissues into the defect [40,41]. The experimental membrane was a GT/PLA barrier membrane with an additive manufacturing process, which has a bionic three-dimensional network structure and is porous, it has an average pore size of 0.2–5.0 μm. The experimental membrane showed good physicochemical properties in the pre-test, with good strength and toughness, tensile strength >1 MPa, and tear strength >1 N. It is easily manipulated, is soft and permeable, and has a good barrier effect without affecting the exchange of small molecules between tissues in the defect area, and the integrity of the experimental membrane can be maintained for at least 4–6 months, which ensures a good bone growth effect. An animal study of GBR in beagle dogs also demonstrated that PLA membranes had good osteogenic capacity, with slower biodegradation and longer spatial maintenance compared to collagen membranes [7].
Although collagen membranes are still the most commonly used material in GBR technology, polymer barrier membranes are gradually becoming not inferior to collagen membranes. In a recent 5-year clinical study, polyethylene glycol membranes were used in GBR and showed similar effectiveness to collagen membranes in terms of implant survival and buccal peri-implant bone and mucosal dimensions after 5 years [42]. The results were similar to those of a 2.5-year clinical study, which compared PLA membranes to collagen membranes, also achieving 100% implant survival. The degree of resorption of the labial bone plate and soft tissue parameters showed that the experimental group was non-inferior to the control group [43]. In another clinical study, 60 implants were placed in the anterior esthetic zone with simultaneous GBR, and the PLA group showed similar results to resorbable collagen membranes in terms of reduction of buccal lateral bone thickness at 4 levels (0 mm, 2 mm, 4 mm, and 6 mm from implant platform to the root) at 6 months. Moreover, osseointegration was achieved with all the implants, proving that implant survival was comparable to the Bio-Gide collagen membrane [44]. The control membrane used in this study (GUIDOR®) was a PLA barrier membrane that has been on the market for more than 20 years and has more experience in clinical use. The results of a 10-year long-term clinical study demonstrated that PLA resorbable barrier membranes in deep 2-wall and 3-wall bone defects achieved good vertical attachment in GTR treatment, and could be stable for up to 10 years postoperatively [45]. The PLA membranes showed comparable results to those of the control group. These indicate that the control membrane in this study has a good long-term clinical outcome and is a satisfactory reference.
To improve the performance of PLA, the PLA membranes in the experimental group were loaded with gelatin components, displaying good biocompatibility and biodegradability [46,47]. Wettability severely influences the material interface and has a profound effect on the bioactivity of barrier membranes. The results confirm that the presence of gelatin improves the hydrophilicity of the membrane due to the tendency of amide groups to form hydrogen bonds with OH in an aqueous environment [48]. In vitro and in vivo studies have shown that GT/PLA has great potential as GBR membranes. Shakeri et al designed GT/PLA membranes with satisfactory tensile strength (4.60±0.14 MPa) and good physicochemical properties, suitable degradation rate, and appropriate porosity (<50%) and pore size. Furthermore, GT/PLA membranes exhibited prolonged antimicrobial drug release with good biocompatibility and cell adhesion [40]. An in vivo beagle dog study of gelatin uniformly attached to PCL membranes showed that the membranes guided the regeneration of new bone and soft tissues at their appropriate sites. The average bone density in the GT/PCL membrane group was approximately 3 times higher than in the GUIDOR® membrane group [49]. Loading gelatin improves the surface hydrophilicity of the PLA, which facilitates cell adhesion and growth. Gelatin has been shown to greatly minimize acid production from PLA degradation, thereby reducing the risk of postoperative complications such as soft tissue fissures or infections in the operative area [47].
Our results showed that the incidence of surgical zone infection was 6.25% (n=1) in both the experimental and control groups. These results are consistent with previous clinical studies of GBR procedures involving a similar duration. A meta-analysis of different barrier membranes in vertically-oriented bone regeneration revealed that PLA membranes had a lower exposure rate [13]. In the present study, no significant membrane exposure was found in the experimental and control groups. In addition, the 2 patients with localized infections exhibited redness and swelling at 2–3 hours postoperatively, which improved with local anti-inflammatory treatment and resulted in successful crown restoration at 6 months. The infection may be related to the localized reaction caused by the acid production of PLA membranes during the degradation process.
Nevertheless, the limitations of the present study should be acknowledged. First, long-term outcomes were not included in our analysis. Although the GUIDOR® membrane, which served as the control group, had favorable long-term clinical and imaging results, the PLA used in the current study has only short-term findings available for reference and there have been few clinical studies on it. Therefore, long-term studies are required to evaluate the long-term biocompatibility and clinical efficacy of the PLA barrier membranes used in this study. Second, one of the study limitations is the low number of study participants in both groups, and the differences in the physical and structural characteristics of the alveolar bone in the maxillary and mandibular regions were not included in the study. Meanwhile, the use of implant systems was not standardized, and heterogeneity in clinical and radiographic results should be interpreted with caution. Finally, histology, histomorphometry, and immunohistochemical analyses were not conducted, all of which constitute major limitations to this study.
Conclusions
In conclusion, the GT/PLA barrier membrane in this study yielded non-inferior clinical and imaging results to the GUIDOR® membrane, and it showed good clinical and image results in GBR techniques. Moreover, the GT/PLA repair membrane exhibited good efficacy and biocompatibility in GBR.
Figures
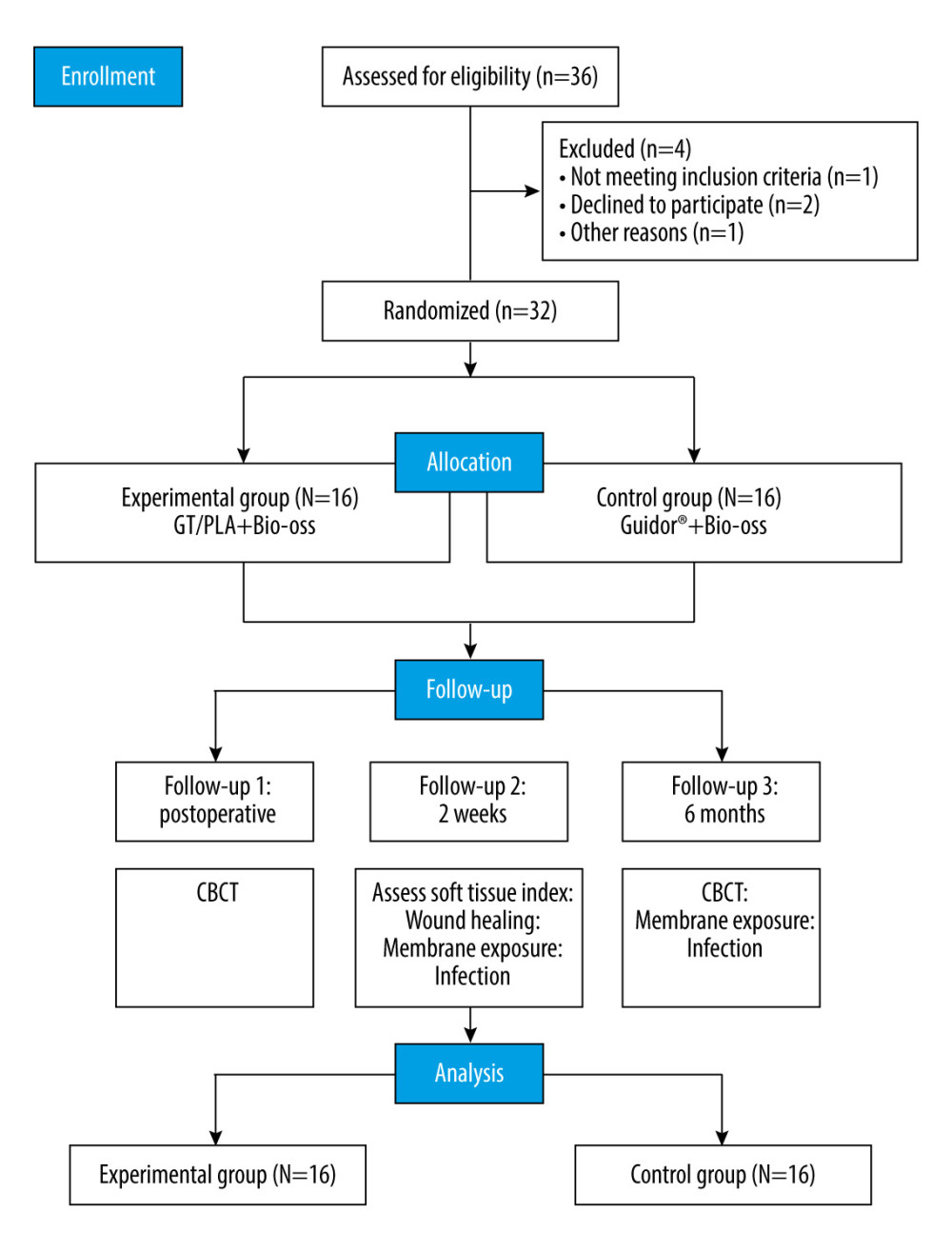
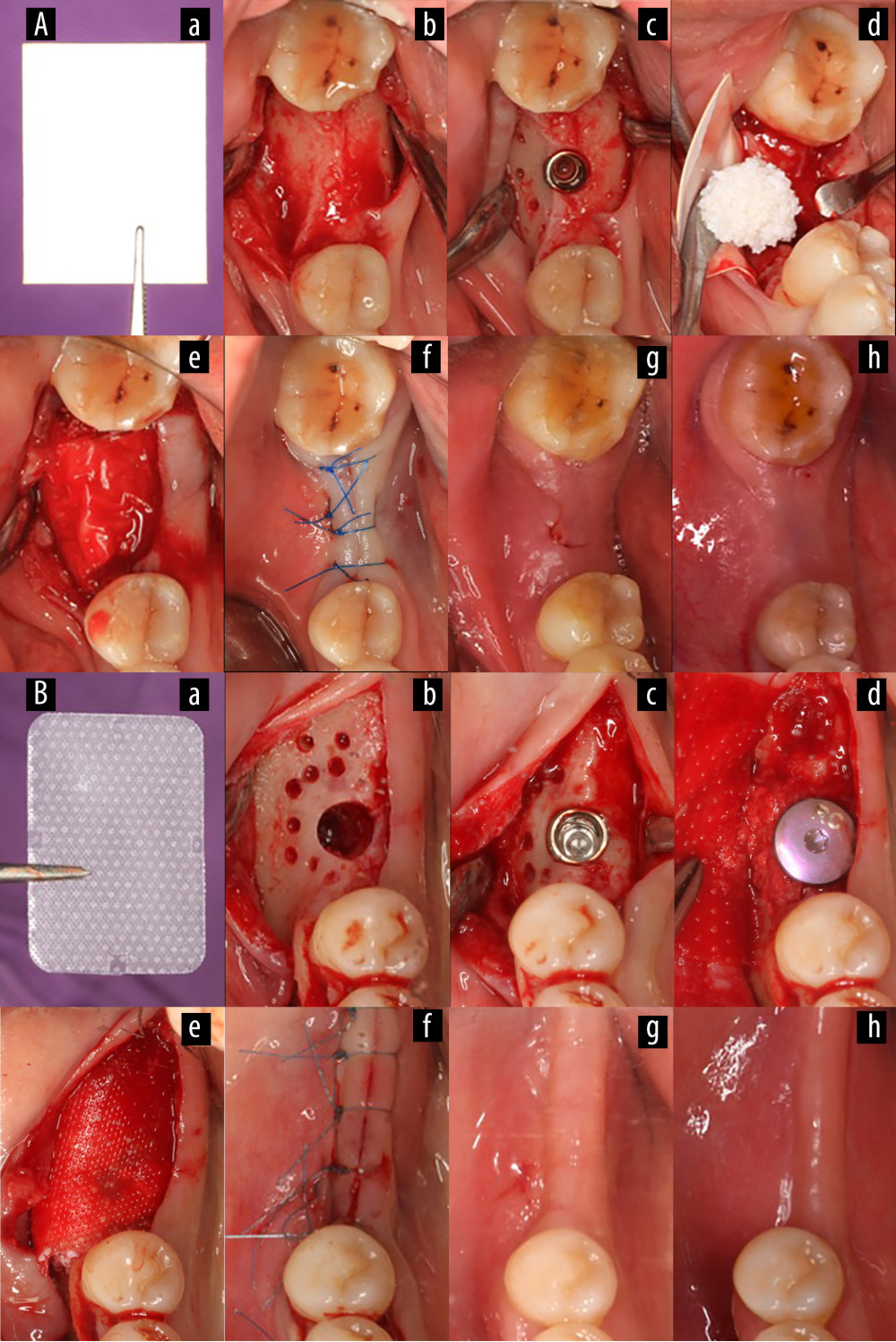
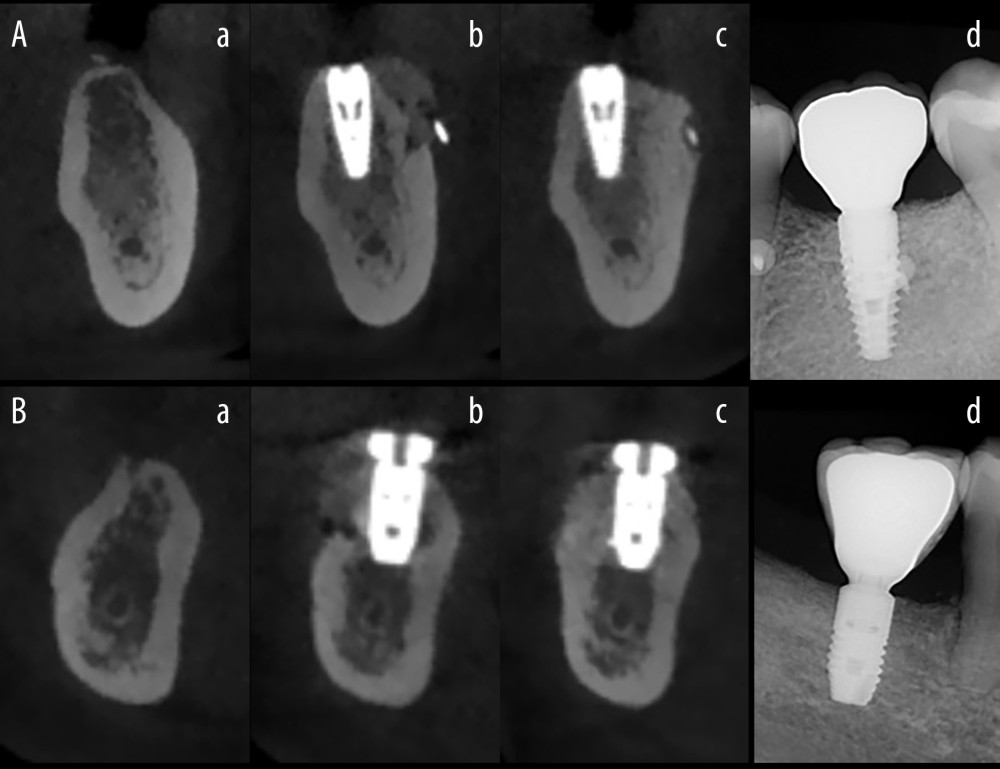
References
1. Tsuchida S, Nakayama T, Recent clinical treatment and basic research on the alveolar bone: Biomedicines, 2023; 11(3); 843
2. Elgali I, Omar O, Dahlin C, Guided bone regeneration: materials and biological mechanisms revisited: Eur J Pharm Sci, 2017; 125(5); 315-37
3. Xue J, Xie J, Liu W, Electrospun nanofibers: New concepts, materials, and applications: Acc Chem Res, 2017; 50(8); 1976-87
4. Di Raimondo R, Sanz-Esporrín J, Plá R, Alveolar crest contour changes after guided bone regeneration using different biomaterials: An experimental in vivo investigation: Clin Oral Investig, 2020; 24(7); 2351-61
5. Jung RE, Brügger LV, Bienz SP, Clinical and radiographical performance of implants placed with simultaneous guided bone regeneration using resorbable and nonresorbable membranes after 22–24 years, a prospective, controlled clinical trial: Clin Oral Implants Res, 2021; 32(12); 1455-65
6. Urban IA, Monje A, Guided bone regeneration in alveolar bone reconstruction: Oral Maxillofac Surg Clin North Am, 2019; 31(2); 331-38
7. Aprile P, Letourneur D, Simon Yarza T, Membranes for guided bone regeneration: A road from bench to bedside: Adv Healthc Mater, 2020; 9(19); 2000707
8. Retzepi M, Donos N, Guided bone regeneration: Biological principle and therapeutic applications: Clin Oral Implants Res, 2010; 21(6); 567-76
9. Donos N, Mardas N, Chadha V, Clinical outcomes of implants following lateral bone augmentation: systematic assessment of available options (barrier membranes, bone grafts, split osteotomy): J Clin Periodontol, 2008; 35(s8); 173-202
10. Teparat T, Solt CW, Claman LJ, Clinical comparison of bioabsorbable barriers with non resorbable barriers in guided tissue regeneration in the treatment of human intrabony defects: J Periodontol, 1998; 69(6); 632-41
11. Guo Y, Su L, Chen C, Clinical efficacy and safety of different dental prosthetic membranes in guided bone regeneration during dental implants: A meta-analysis: Comput Intell Neurosci, 2022; 2022; 3245014
12. Wallkamm B, Schmid J, Hämmerle CHF: Clin Oral Implants Res, 2003; 14(6); 734-42
13. Zhang M, Zhou Z, Yun J, Effect of different membranes on vertical bone regeneration: A systematic review and network meta-analysis: Biomed Res Int, 2022; 2022; 7742687
14. Di Raimondo R, Sanz-Esporrín J, Sanz-Martin I, Hard and soft tissue changes after guided bone regeneration using two different barrier membranes: An experimental in vivo investigation: Clin Oral Investig, 2021; 25(4); 2213-27
15. Mizraji G, Davidzohn A, Gursoy M, Membrane barriers for guided bone regeneration: An overview of available biomaterials: Periodontology 2000, 2023; 93(1); 56-76
16. Ren Y, Fan L, Alkildani S, Barrier membranes for guided bone regeneration (GBR): A focus on recent advances in collagen membranes: Int J Mol Sci, 2022; 23(23); 14987
17. Jung RE, Herzog M, Wolleb K, A randomized controlled clinical trial comparing small buccal dehiscence defects around dental implants treated with guided bone regeneration or left for spontaneous healing: Clin Oral Implants Res, 2017; 28(3); 348-54
18. Liu SQ, Zheng YY, Liu RL, Preparation and characterization of a novel polylactic acid/hydroxyapatite composite scaffold with biomimetic micro-nanofibrous porous structure: J Mater Sci Mater Med, 2020; 31(8); 74
19. Radwan-Praglowska J, Janus L, Piatkowski M: Polymers, 2020; 12(4); 792
20. Gunjiganur Vemanaradhya DG, Rahman DS, Adi DDM, Impact of glutaraldehyde cross-linking on the advanced platelet-rich fibrin membrane: Macroscopic, conventional light microscopic and scanning electron microscopic analysis: Dent Med Probl, 2023; 60(1); 79-86
21. Chu C, Wang Y, Wang Y, Evaluation of epigallocatechin-3-gallate (EGCG) modified collagen in guided bone regeneration (GBR) surgery and modulation of macrophage phenotype: Mater Sci Eng C Mater Biol Appl, 2019; 99; 73-82
22. Urban IA, Wessing B, Alandez N, A multicenter randomized controlled trial using a novel collagen membrane for guided bone regeneration at dehisced single implant sites: Outcome at prosthetic delivery and at 1-year follow-up: Clin Oral Implants Res, 2019; 30(6); 487-97
23. Jung RE, Fenner N, Hämmerle CHF, Long term outcome of implants placed with guided bone regeneration (GBR) using resorbable and non resorbable membranes after 12–14 years: Clin Oral Implants Res, 2013; 24(10); 1065-73
24. Chen H, Müller MB, Gilmore KJ, Mechanically strong, electrically conductive, and biocompatible graphene paper: Adv Mater, 2008; 20(18); 3557-61
25. Abdelaziz D, Hefnawy A, Al-Wakeel E, New biodegradable nanoparticles-in-nanofibers based membranes for guided periodontal tissue and bone regeneration with enhanced antibacterial activity: J Adv Res, 2021; 28; 51-62
26. De Moura NK, Martins EF, Oliveira RLMS, Synergistic effect of adding bioglass and carbon nanotubes on poly (lactic acid) porous membranes for guided bone regeneration: Mater Sci Eng: C, 2020; 117; 111327
27. Hwang C, Park S, Kang I-G, Tantalum-coated polylactic acid fibrous membranes for guided bone regeneration: Mater Sci Eng: C, 2020; 115; 111112
28. Polimeni G, Koo KT, Pringle GA, Histopathological observations of a polylactic acid-based device intended for guided bone/tissue regeneration: Clin Implant Dent Relat Res, 2008; 10(2); 99-105
29. Rüdiger SG, Ehmke B, Hommens A, Guided tissue regeneration using a polylactic acid barrier. Part I: Environmental effects on bacterial colonization: J Clin Periodontol, 2003; 30(1); 19-25
30. Kawasaki T, Ohba S, Nakatani Y, Clinical study of guided bone regeneration with resorbable polylactide-co-glycolide acid membrane: Odontology, 2018; 106(3); 334-39
31. Wang YT, Zhang X, Zhang S, Development of a rapid-shaping and user-friendly membrane with long-lasting space maintenance for guided bone regeneration: J Mater Chem B, 2024; 12(6); 1495-511
32. Li B, Chen Y, He J, Silk fibroin/methacrylated gelatine/hydroxyapatite biomimetic nanofibrous membranes for guided bone regeneration: Int J Biol Macromol, 2024; 263(Pt 2); 130380
33. He Y, Jin Y, Wang X, An antimicrobial peptide-loaded gelatin/chitosan nanofibrous membrane fabricated by sequential layer-by-layer electrospinning and electrospraying techniques: Nanomaterials, 2018; 8(5); 327
34. Joo G, Park M, Park SS, Tailored alginate/PCL-gelatin-β-TCP membrane for guided bone regeneration: Biomed Mater, 2022; 17(4); 1748-605X/ac6bd8
35. Casalini T, Rossi F, Castrovinci A, A Perspective on polylactic acid-based polymers use for nanoparticles synthesis and applications: Front Bioeng Biotechnol, 2019; 7; 259
36. Deng K, Yang Y, Ke Y, A novel biomimetic composite substitute of PLLA/gelatin nanofiber membrane for dura repairing: Neurol Res, 2017; 39(9); 819-29
37. Karapataki S, Hugoson A, Falk H: J Clin Periodontol, 2000; 27(5); 333-40
38. Zhang W, Wang N, Yang M, Periosteum and development of the tissue-engineered periosteum for guided bone regeneration: J Orthop Translat, 2022; 33; 41-54
39. Zhang HY, Jiang HB, Ryu J-H, Comparing properties of variable pore-sized 3D-printed PLA membrane with conventional PLA membrane for guided bone/tissue regeneration: Materials, 2019; 12(10); 1718
40. Shakeri H, Haghbin Nazarpak M, Imani R, Poly (l-lactic acid)-based modified nanofibrous membrane with dual drug release capability for GBR application: Int J Biol Macromol, 2023; 231; 123201
41. Shu Z, Zhang C, Yan L, Antibacterial and osteoconductive polycaprolactone/polylactic acid/nano-hydroxyapatite/Cu@ZIF-8 GBR membrane with asymmetric porous structure: Int J Biol Macromol, 2023; 224; 1040-51
42. Jung RE, Benic GI, Scherrer D, Cone beam computed tomography evaluation of regenerated buccal bone 5 years after simultaneous implant placement and guided bone regeneration procedures – a randomized, controlled clinical trial: Clin Oral Implants Res, 2015; 26(1); 28-34
43. Li X, Jin Q, Xu H, Effect of polylactic acid membrane on guided bone regeneration in anterior maxillary implantation: Med Sci Monit, 2023; 29; e938566
44. Arunjaroensuk S, Panmekiate S, Pimkhaokham A, The stability of augmented bone between two different membranes used for guided bone regeneration simultaneous with dental implant placement in the esthetic zone: Int J Oral Maxillofac Implants, 2018; 33(1); 206-16
45. Nickles K, Ratka Krüger P, Neukranz E, Open flap debridement and guided tissue regeneration after 10 years in infrabony defects: J Clin Periodontol, 2009; 36(11); 976-83
46. Malekahmadi B, Esfahanian V, Ejeian F, In vitro study of the recruitment and expansion of mesenchymal stem cells at the interface of a Cu-doped PCL-bioglass scaffold: Biomimetics, 2022; 7(1); 19
47. Song J-H, Kim H-E, Kim H-W, Production of electrospun gelatin nanofiber by water-based co-solvent approach: J Mater Sci Mater Med, 2008; 19(1); 95-102
48. Lyu S, Huang C, Yang H, Electrospun fibers as a scaffolding platform for bone tissue repair: J Orthop Res, 2013; 31(9); 1382-89
49. Jamalpour MR, Yadegari A, Vahdatinia F, 3D-printed bi-layered polymer/hydrogel construct for interfacial tissue regeneration in a canine model: Dent Mater, 2022; 38(8); 1316-29
Figures
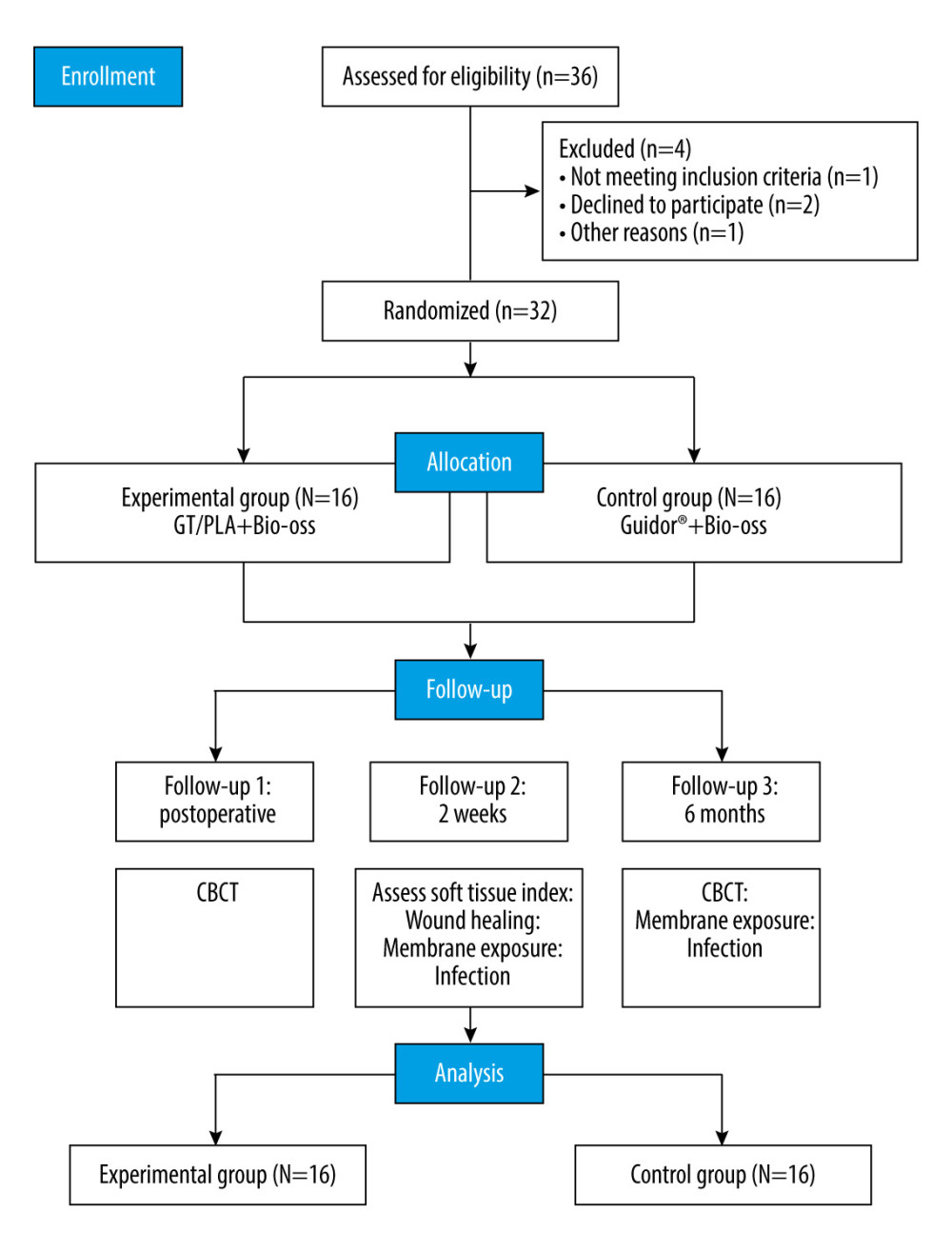
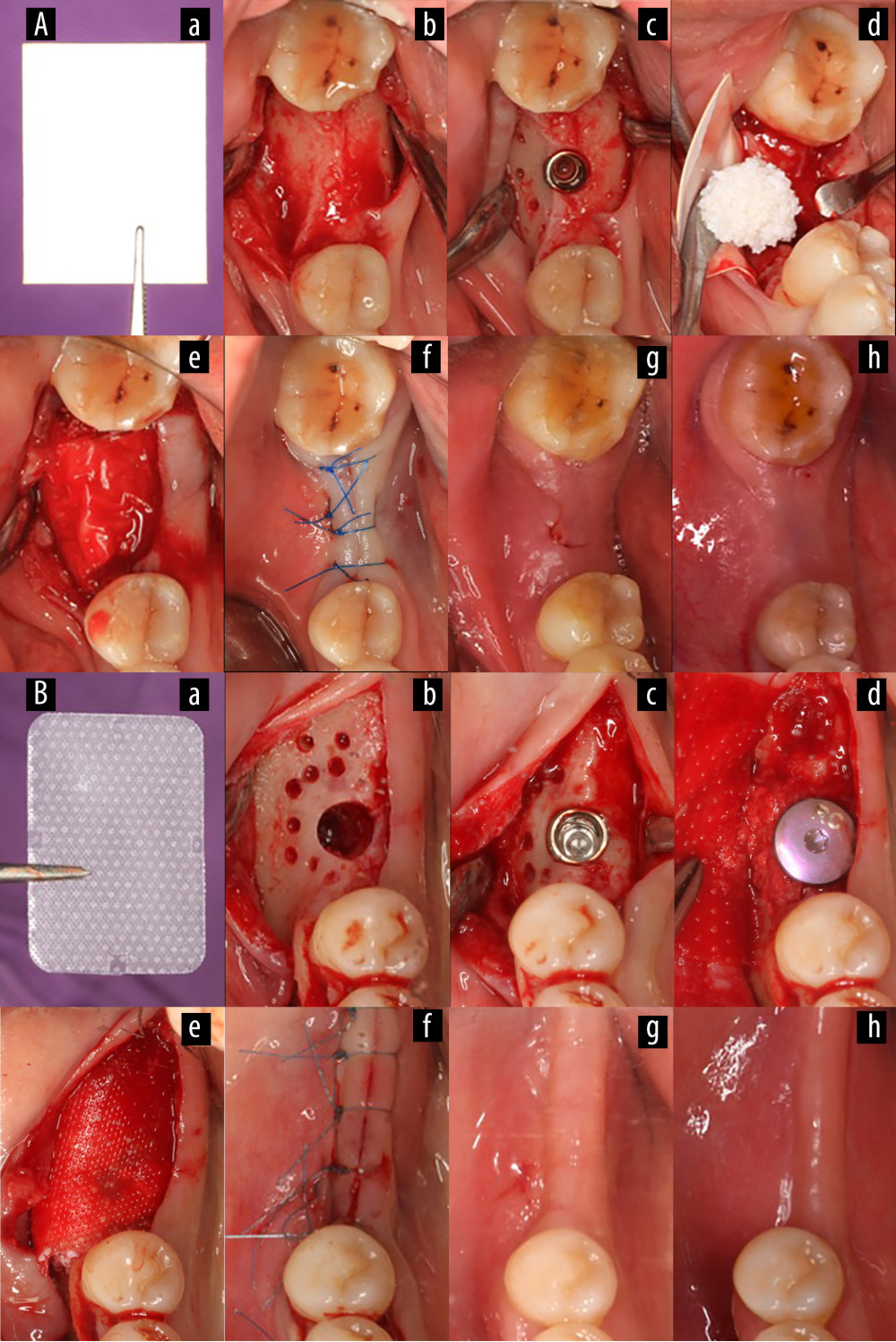
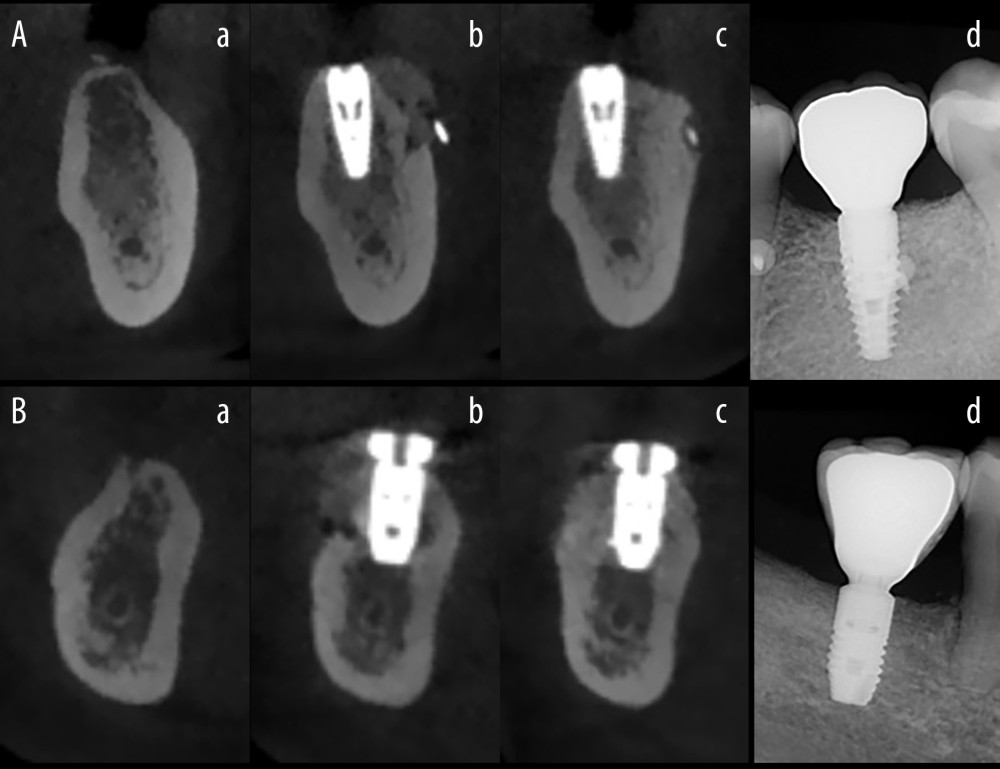
Tables
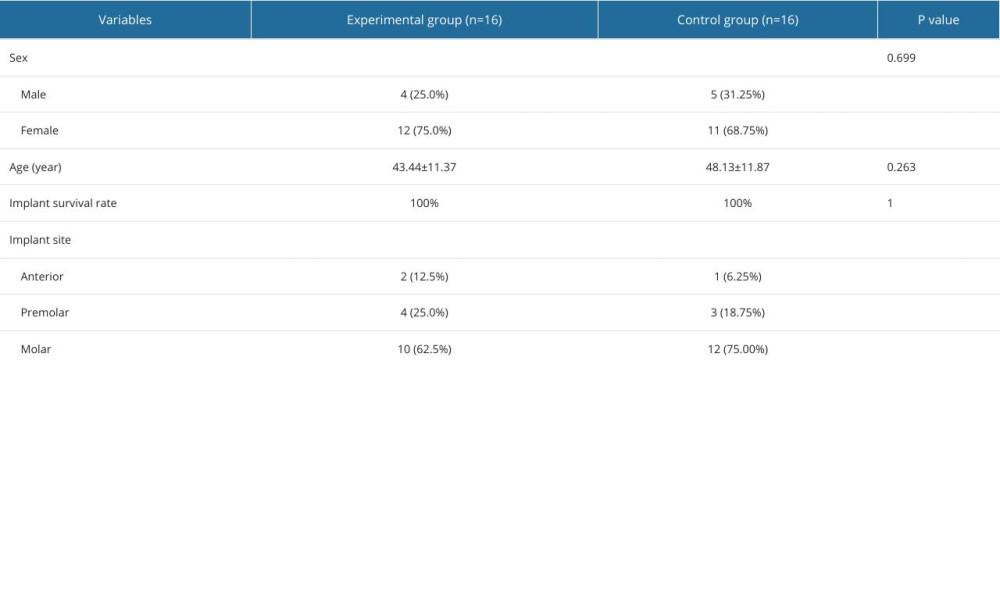
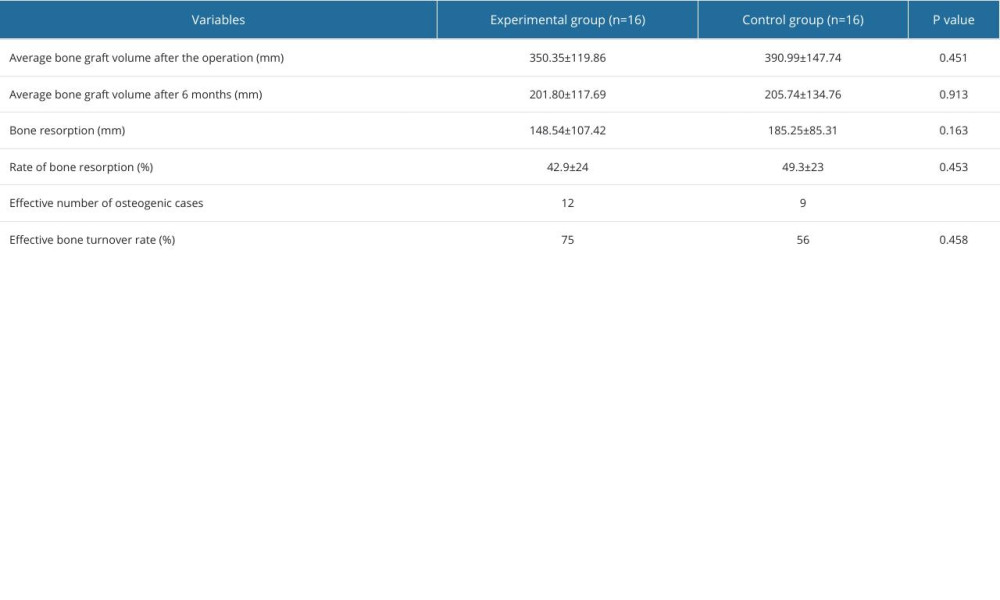
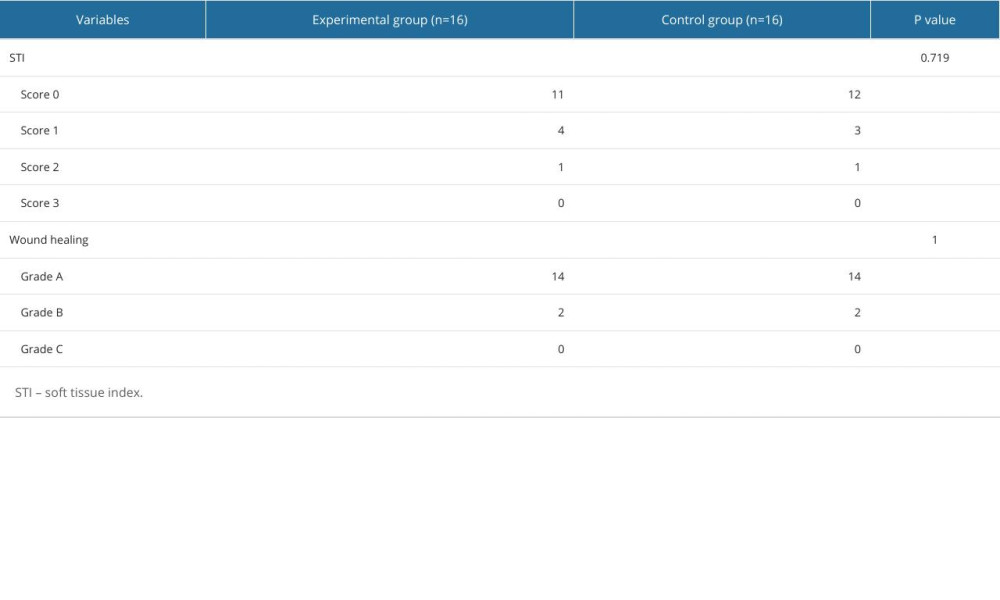
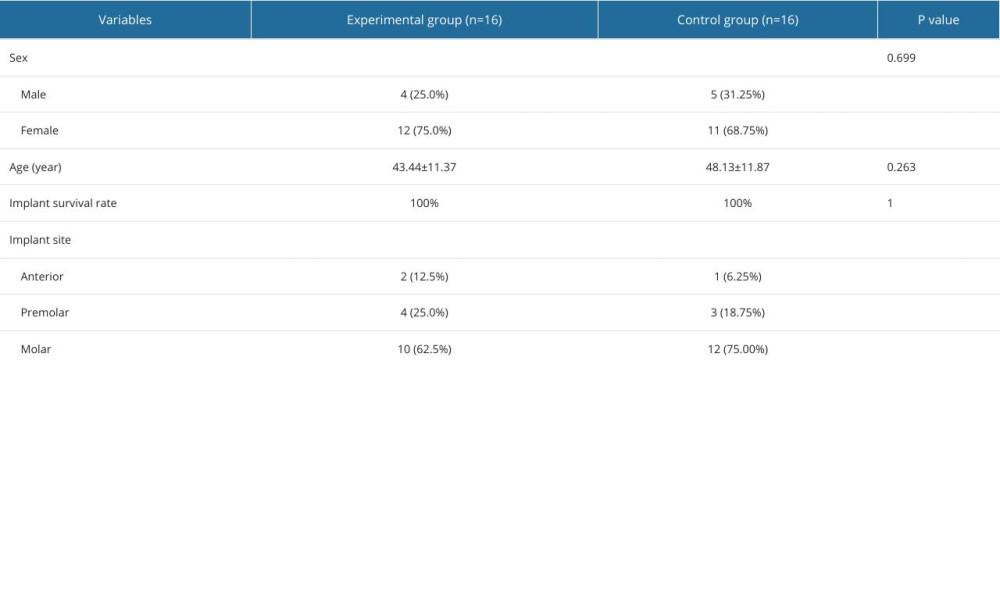
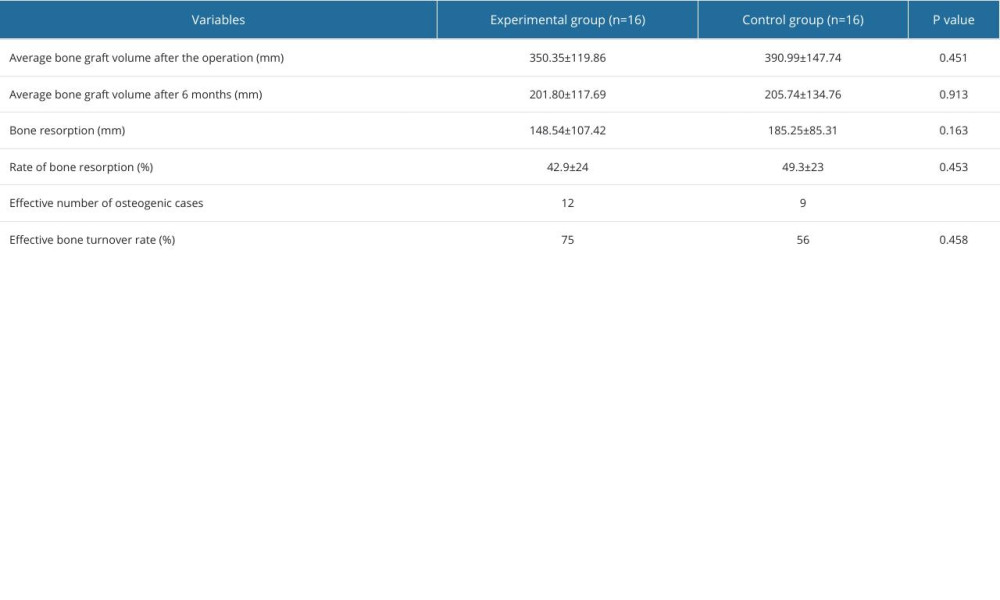
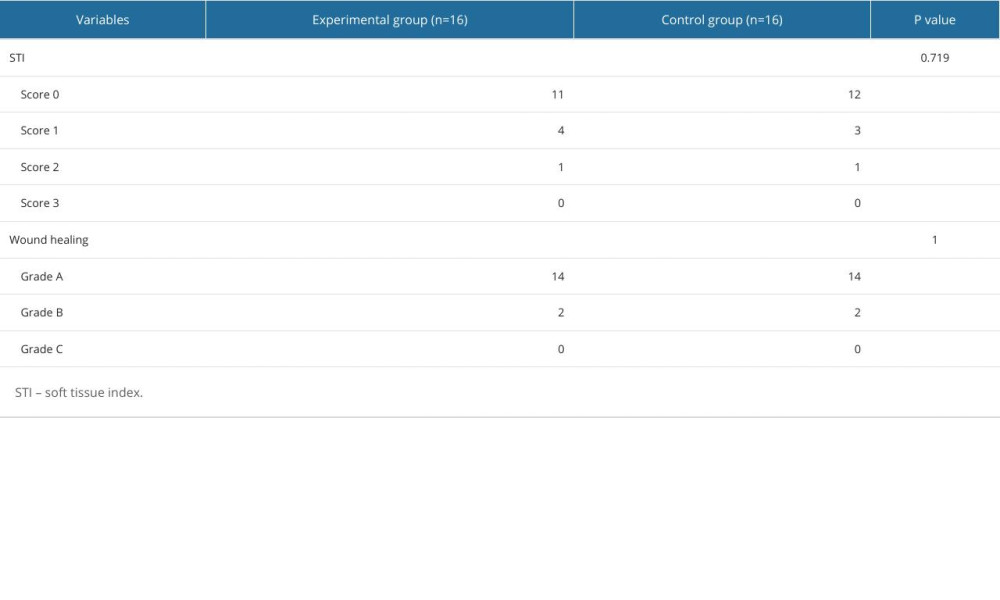
In Press
Clinical Research
Assessing Caries Removal Efficacy and Pain Perception in Children, Using Smart Bur Versus Carbide Bur: A Ra...Med Sci Monit In Press; DOI: 10.12659/MSM.946802
Clinical Research
Chiari Malformation Type I and Syringomyelia: Outcomes of Arachnoid-Preserving Surgical TechniqueMed Sci Monit In Press; DOI: 10.12659/MSM.946978
Animal Research
Role of the Dorsal Cortex of the Inferior Colliculus in the Precedence EffectMed Sci Monit In Press; DOI: 10.12659/MSM.945605
Laboratory Research
Comparative Evaluation of the Dimensional Accuracy of Silicone-Based Putty Reline Impressions with Differen...Med Sci Monit In Press; DOI: 10.12659/MSM.946537
Most Viewed Current Articles
17 Jan 2024 : Review article 6,961,910
Vaccination Guidelines for Pregnant Women: Addressing COVID-19 and the Omicron VariantDOI :10.12659/MSM.942799
Med Sci Monit 2024; 30:e942799
16 May 2023 : Clinical Research 699,502
Electrophysiological Testing for an Auditory Processing Disorder and Reading Performance in 54 School Stude...DOI :10.12659/MSM.940387
Med Sci Monit 2023; 29:e940387
01 Mar 2024 : Editorial 22,584
Editorial: First Regulatory Approvals for CRISPR-Cas9 Therapeutic Gene Editing for Sickle Cell Disease and ...DOI :10.12659/MSM.944204
Med Sci Monit 2024; 30:e944204
28 Jan 2024 : Review article 17,437
A Review of IgA Vasculitis (Henoch-Schönlein Purpura) Past, Present, and FutureDOI :10.12659/MSM.943912
Med Sci Monit 2024; 30:e943912