AKIR-1 regulates proteasome subcellular function in Caenorhabditis elegans
- PMID: 37767001
- PMCID: PMC10520889
- DOI: 10.1016/j.isci.2023.107886
AKIR-1 regulates proteasome subcellular function in Caenorhabditis elegans
Abstract
Polyubiquitinated proteins are primarily degraded by the ubiquitin-proteasome system (UPS). Proteasomes are present both in the cytoplasm and nucleus. Here, we investigated mechanisms coordinating proteasome subcellular localization and activity in a multicellular organism. We identified the nuclear protein-encoding gene akir-1 as a proteasome regulator in a genome-wide Caenorhabditis elegans RNAi screen. We demonstrate that depletion of akir-1 causes nuclear accumulation of endogenous polyubiquitinated proteins in intestinal cells, concomitant with slower in vivo proteasomal degradation in this subcellular compartment. Remarkably, akir-1 is essential for nuclear localization of proteasomes both in oocytes and intestinal cells but affects differentially the subcellular distribution of polyubiquitinated proteins. We further reveal that importin ima-3 genetically interacts with akir-1 and influences nuclear localization of a polyubiquitin-binding reporter. Our study shows that the conserved AKIR-1 is an important regulator of the subcellular function of proteasomes in a multicellular organism, suggesting a role for AKIR-1 in proteostasis maintenance.
Keywords: Biochemistry; Cell biology; Genomics.
© 2023 The Author(s).
Conflict of interest statement
The authors declare no competing interests.
Figures
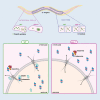
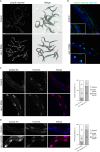
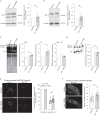
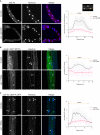
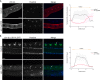
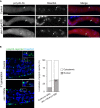
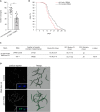
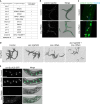
Similar articles
-
A Novel Role for α-Importins and Akirin in Establishment of Meiotic Sister Chromatid Cohesion in Caenorhabditis elegans.Genetics. 2019 Feb;211(2):617-635. doi: 10.1534/genetics.118.301458. Epub 2018 Dec 18. Genetics. 2019. PMID: 30563860 Free PMC article.
-
Fluorescent Tools for In Vivo Studies on the Ubiquitin-Proteasome System.Methods Mol Biol. 2016;1449:215-22. doi: 10.1007/978-1-4939-3756-1_12. Methods Mol Biol. 2016. PMID: 27613038
-
Tissue-Specific Impact of Autophagy Genes on the Ubiquitin-Proteasome System in C. elegans.Cells. 2020 Aug 8;9(8):1858. doi: 10.3390/cells9081858. Cells. 2020. PMID: 32784405 Free PMC article.
-
Intracellular localization of the proteasome in response to stress conditions.J Biol Chem. 2022 Jul;298(7):102083. doi: 10.1016/j.jbc.2022.102083. Epub 2022 May 27. J Biol Chem. 2022. PMID: 35636514 Free PMC article. Review.
-
Nuclear Transport of Yeast Proteasomes.Front Mol Biosci. 2019 May 16;6:34. doi: 10.3389/fmolb.2019.00034. eCollection 2019. Front Mol Biosci. 2019. PMID: 31157235 Free PMC article. Review.
Cited by
-
Research progress on oncoprotein hepatitis B X‑interacting protein (Review).Mol Med Rep. 2024 Jun;29(6):89. doi: 10.3892/mmr.2024.13213. Epub 2024 Apr 5. Mol Med Rep. 2024. PMID: 38577934 Free PMC article. Review.