Mrs2p is an essential component of the major electrophoretic Mg2+ influx system in mitochondria
- PMID: 12628916
- PMCID: PMC151051
- DOI: 10.1093/emboj/cdg122
Mrs2p is an essential component of the major electrophoretic Mg2+ influx system in mitochondria
Abstract
Steady-state concentrations of mitochondrial Mg(2+) previously have been shown to vary with the expression of Mrs2p, a component of the inner mitochondrial membrane with two transmembrane domains. While its structural and functional similarity to the bacterial Mg(2+) transport protein CorA suggested a role for Mrs2p in Mg(2+) influx into the organelle, other functions in cation homeostasis could not be excluded. Making use of the fluorescent dye mag-fura 2 to measure free Mg(2+) concentrations continuously, we describe here a high capacity, rapid Mg(2+) influx system in isolated yeast mitochondria, driven by the mitochondrial membrane potential Deltapsi and inhibited by cobalt(III)hexaammine. Overexpression of Mrs2p increases influx rates 5-fold, while the deletion of the MRS2 gene abolishes this high capacity Mg(2+) influx. Mg(2+) efflux from isolated mitochondria, observed with low Deltapsi only, also requires the presence of Mrs2p. Cross-linking experiments revealed the presence of Mrs2p-containing complexes in the mitochondrial membrane, probably constituting Mrs2p homo- oligomers. Taken together, these findings characterize Mrs2p as the first molecularly identified metal ion channel protein in the inner mitochondrial membrane.
Figures
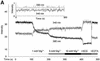
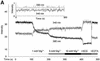
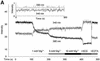
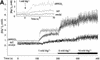
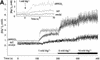
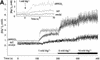
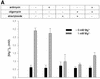
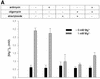
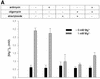
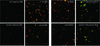
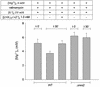
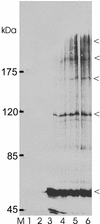
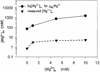
Similar articles
-
Lpe10p modulates the activity of the Mrs2p-based yeast mitochondrial Mg2+ channel.FEBS J. 2010 Sep;277(17):3514-25. doi: 10.1111/j.1742-4658.2010.07761.x. Epub 2010 Jul 23. FEBS J. 2010. PMID: 20653776
-
Mutational analysis of functional domains in Mrs2p, the mitochondrial Mg2+ channel protein of Saccharomyces cerevisiae.FEBS J. 2006 Mar;273(6):1198-209. doi: 10.1111/j.1742-4658.2006.05157.x. FEBS J. 2006. PMID: 16519685
-
The bacterial magnesium transporter CorA can functionally substitute for its putative homologue Mrs2p in the yeast inner mitochondrial membrane.J Biol Chem. 1999 Jul 16;274(29):20438-43. doi: 10.1074/jbc.274.29.20438. J Biol Chem. 1999. PMID: 10400670
-
The enigmatic role of Mim1 in mitochondrial biogenesis.Eur J Cell Biol. 2010 Feb-Mar;89(2-3):212-5. doi: 10.1016/j.ejcb.2009.11.002. Epub 2009 Nov 26. Eur J Cell Biol. 2010. PMID: 19944477 Review.
-
Magnesium transporters: properties, regulation and structure.Front Biosci. 2006 Sep 1;11:3149-63. doi: 10.2741/2039. Front Biosci. 2006. PMID: 16720382 Review.
Cited by
-
Positional cloning of rat mutant genes reveals new functions of these genes.Exp Anim. 2023 Feb 21;72(1):1-8. doi: 10.1538/expanim.22-0089. Epub 2022 Sep 3. Exp Anim. 2023. PMID: 36058846 Free PMC article. Review.
-
Impact of intracellular ion channels on cancer development and progression.Eur Biophys J. 2016 Oct;45(7):685-707. doi: 10.1007/s00249-016-1143-0. Epub 2016 Jun 11. Eur Biophys J. 2016. PMID: 27289382 Free PMC article. Review.
-
Fluorescent Indicators For Biological Imaging of Monatomic Ions.Front Cell Dev Biol. 2022 Apr 27;10:885440. doi: 10.3389/fcell.2022.885440. eCollection 2022. Front Cell Dev Biol. 2022. PMID: 35573682 Free PMC article. Review.
-
Structural and functional comparison of magnesium transporters throughout evolution.Cell Mol Life Sci. 2022 Jul 12;79(8):418. doi: 10.1007/s00018-022-04442-8. Cell Mol Life Sci. 2022. PMID: 35819535 Free PMC article. Review.
-
Functional analysis of the conserved hydrophobic gate region of the magnesium transporter CorA.Biochim Biophys Acta. 2011 Jun;1808(6):1587-91. doi: 10.1016/j.bbamem.2010.10.017. Epub 2010 Nov 11. Biochim Biophys Acta. 2011. PMID: 21074514 Free PMC article.
References
-
- Baumann F., Neupert,W. and Herrmann,J.M. (2002) Insertion of bitopic membrane proteins into the inner membrane of mitochondria involves an export step from the matrix. J. Biol. Chem., 277, 21405–21413. - PubMed
-
- Bui D.M., Gregan,J., Jarosch,E., Ragnini,A. and Schweyen,R.J. (1999) The bacterial Mg2+ transporter CorA can functionally substitute for its putative homologue Mrs2p in the yeast inner mitochondrial membrane. J. Biol. Chem., 274, 20438–20443. - PubMed
-
- Diwan J.J. (1987) Mitochondrial transport of K+ and Mg2+. Biochim. Biophys. Acta, 895, 155–165. - PubMed
-
- Diwan J.J., Daze,M., Richardson,R. and Aronson,D. (1979) Kinetics of Mg2+ flux into rat liver mitochondria. Biochemistry, 18, 2590–2595. - PubMed
-
- Graschopf A., Stadler,J.A., Hoellerer,M.K., Eder,S., Sieghardt.M., Kohlwein,S.D. and Schweyen R.J. (2001) The yeast plasma membrane protein Alr1 controls Mg2+ homeostasis and is subject to Mg2+-dependent control of its synthesis and degradation. J. Biol. Chem., 276, 16216–16222. - PubMed
Publication types
MeSH terms
Substances
LinkOut - more resources
Full Text Sources
Other Literature Sources
Molecular Biology Databases
Research Materials