An adenosine-to-inosine tRNA-editing enzyme that can perform C-to-U deamination of DNA
- PMID: 17483465
- PMCID: PMC1876531
- DOI: 10.1073/pnas.0702394104
An adenosine-to-inosine tRNA-editing enzyme that can perform C-to-U deamination of DNA
Abstract
Adenosine-to-inosine editing in the anticodon of tRNAs is essential for viability. Enzymes mediating tRNA adenosine deamination in bacteria and yeast contain cytidine deaminase-conserved motifs, suggesting an evolutionary link between the two reactions. In trypanosomatids, tRNAs undergo both cytidine-to-uridine and adenosine-to-inosine editing, but the relationship between the two reactions is unclear. Here we show that down-regulation of the Trypanosoma brucei tRNA-editing enzyme by RNAi leads to a reduction in both C-to-U and A-to-I editing of tRNA in vivo. Surprisingly, in vitro, this enzyme can mediate A-to-I editing of tRNA and C-to-U deamination of ssDNA but not both in either substrate. The ability to use both DNA and RNA provides a model for a multispecificity editing enzyme. Notably, the ability of a single enzyme to perform two different deamination reactions also suggests that this enzyme still maintains specificities that would have been found in the ancestor deaminase, providing a first line of evidence for the evolution of editing deaminases.
Conflict of interest statement
The authors declare no conflict of interest.
Figures
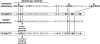
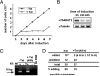
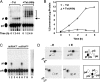
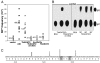
Similar articles
-
Identification of enzymes for adenosine-to-inosine editing and discovery of cytidine-to-uridine editing in nucleus-encoded transfer RNAs of Arabidopsis.Plant Physiol. 2014 Dec;166(4):1985-97. doi: 10.1104/pp.114.250498. Epub 2014 Oct 14. Plant Physiol. 2014. PMID: 25315605 Free PMC article.
-
Identification of the chloroplast adenosine-to-inosine tRNA editing enzyme.RNA. 2009 Jul;15(7):1251-7. doi: 10.1261/rna.1600609. Epub 2009 May 21. RNA. 2009. PMID: 19460869 Free PMC article.
-
A-to-I editing on tRNAs: biochemical, biological and evolutionary implications.FEBS Lett. 2014 Nov 28;588(23):4279-86. doi: 10.1016/j.febslet.2014.09.025. Epub 2014 Sep 27. FEBS Lett. 2014. PMID: 25263703 Review.
-
RNA editing by base deamination: more enzymes, more _targets, new mysteries.Trends Biochem Sci. 2001 Jun;26(6):376-84. doi: 10.1016/s0968-0004(01)01827-8. Trends Biochem Sci. 2001. PMID: 11406411 Review.
-
Mechanism, specificity and general properties of the yeast enzyme catalysing the formation of inosine 34 in the anticodon of transfer RNA.J Mol Biol. 1996 Oct 4;262(4):437-58. doi: 10.1006/jmbi.1996.0527. J Mol Biol. 1996. PMID: 8893855
Cited by
-
C to U editing at position 32 of the anticodon loop precedes tRNA 5' leader removal in trypanosomatids.Nucleic Acids Res. 2007;35(20):6740-9. doi: 10.1093/nar/gkm745. Epub 2007 Oct 4. Nucleic Acids Res. 2007. PMID: 17916576 Free PMC article.
-
Formation of tRNA Wobble Inosine in Humans Is Disrupted by a Millennia-Old Mutation Causing Intellectual Disability.Mol Cell Biol. 2019 Sep 11;39(19):e00203-19. doi: 10.1128/MCB.00203-19. Print 2019 Oct 1. Mol Cell Biol. 2019. PMID: 31263000 Free PMC article.
-
The Importance of ncRNAs as Epigenetic Mechanisms in Phenotypic Variation and Organic Evolution.Front Microbiol. 2017 Dec 22;8:2483. doi: 10.3389/fmicb.2017.02483. eCollection 2017. Front Microbiol. 2017. PMID: 29312192 Free PMC article. Review.
-
Comparative genomic characterization of Francisella tularensis strains belonging to low and high virulence subspecies.PLoS Pathog. 2009 May;5(5):e1000459. doi: 10.1371/journal.ppat.1000459. Epub 2009 May 29. PLoS Pathog. 2009. PMID: 19478886 Free PMC article.
-
Non coding RNAs and viruses in the framework of the phylogeny of the genes, epigenesis and heredity.Int J Mol Sci. 2012;13(1):477-490. doi: 10.3390/ijms13010477. Epub 2012 Jan 4. Int J Mol Sci. 2012. PMID: 22312265 Free PMC article. Review.
References
MeSH terms
Substances
Grants and funding
LinkOut - more resources
Full Text Sources
Other Literature Sources
Molecular Biology Databases
Research Materials