The checkpoint Saccharomyces cerevisiae Rad9 protein contains a tandem tudor domain that recognizes DNA
- PMID: 17726056
- PMCID: PMC2034471
- DOI: 10.1093/nar/gkm607
The checkpoint Saccharomyces cerevisiae Rad9 protein contains a tandem tudor domain that recognizes DNA
Abstract
DNA damage checkpoints are signal transduction pathways that are activated after genotoxic insults to protect genomic integrity. At the site of DNA damage, 'mediator' proteins are in charge of recruiting 'signal transducers' to molecules 'sensing' the damage. Budding yeast Rad9, fission yeast Crb2 and metazoan 53BP1 are presented as mediators involved in the activation of checkpoint kinases. Here we show that, despite low sequence conservation, Rad9 exhibits a tandem tudor domain structurally close to those found in human/mouse 53BP1 and fission yeast Crb2. Moreover, this region is important for the resistance of Saccharomyces cerevisiae to different genotoxic stresses. It does not mediate direct binding to a histone H3 peptide dimethylated on K79, nor to a histone H4 peptide dimethylated on lysine 20, as was demonstrated for 53BP1. However, the tandem tudor region of Rad9 directly interacts with single-stranded DNA and double-stranded DNAs of various lengths and sequences through a positively charged region absent from 53BP1 and Crb2 but present in several yeast Rad9 homologs. Our results argue that the tandem tudor domains of Rad9, Crb2 and 53BP1 mediate chromatin binding next to double-strand breaks. However, their modes of chromatin recognition are different, suggesting that the corresponding interactions are differently regulated.
Figures
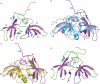
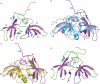
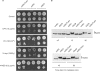
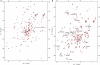
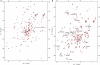
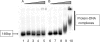
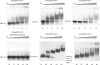
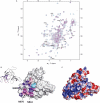
Similar articles
-
Boundaries and physical characterization of a new domain shared between mammalian 53BP1 and yeast Rad9 checkpoint proteins.Protein Sci. 2005 Jul;14(7):1827-39. doi: 10.1110/ps.041305205. Protein Sci. 2005. PMID: 15987907 Free PMC article.
-
Role of Dot1-dependent histone H3 methylation in G1 and S phase DNA damage checkpoint functions of Rad9.Mol Cell Biol. 2005 Oct;25(19):8430-43. doi: 10.1128/MCB.25.19.8430-8443.2005. Mol Cell Biol. 2005. PMID: 16166626 Free PMC article.
-
Methylated lysine 79 of histone H3 _targets 53BP1 to DNA double-strand breaks.Nature. 2004 Nov 18;432(7015):406-11. doi: 10.1038/nature03114. Epub 2004 Nov 3. Nature. 2004. PMID: 15525939
-
ATM signaling and 53BP1.Radiother Oncol. 2005 Aug;76(2):119-22. doi: 10.1016/j.radonc.2005.06.026. Radiother Oncol. 2005. PMID: 16024119 Review.
-
Role of the Saccharomyces cerevisiae Rad9 protein in sensing and responding to DNA damage.Biochem Soc Trans. 2003 Feb;31(Pt 1):242-6. doi: 10.1042/bst0310242. Biochem Soc Trans. 2003. PMID: 12546694 Review.
Cited by
-
An oligomerized 53BP1 tudor domain suffices for recognition of DNA double-strand breaks.Mol Cell Biol. 2009 Feb;29(4):1050-8. doi: 10.1128/MCB.01011-08. Epub 2008 Dec 8. Mol Cell Biol. 2009. PMID: 19064641 Free PMC article.
-
Chromatin remodeling finds its place in the DNA double-strand break response.Nucleic Acids Res. 2009 Apr;37(5):1363-77. doi: 10.1093/nar/gkn1071. Epub 2009 Jan 12. Nucleic Acids Res. 2009. PMID: 19139074 Free PMC article. Review.
-
Mad2 prolongs DNA damage checkpoint arrest caused by a double-strand break via a centromere-dependent mechanism.Curr Biol. 2010 Feb 23;20(4):328-32. doi: 10.1016/j.cub.2009.12.033. Epub 2010 Jan 21. Curr Biol. 2010. PMID: 20096585 Free PMC article.
-
Inviability of a DNA2 deletion mutant is due to the DNA damage checkpoint.Cell Cycle. 2011 May 15;10(10):1690-8. doi: 10.4161/cc.10.10.15643. Epub 2011 May 15. Cell Cycle. 2011. PMID: 21508669 Free PMC article.
-
The structure of a highly-conserved picocyanobacterial protein reveals a Tudor domain with an RNA-binding function.J Biol Chem. 2019 Sep 27;294(39):14333-14344. doi: 10.1074/jbc.RA119.007938. Epub 2019 Aug 7. J Biol Chem. 2019. PMID: 31391250 Free PMC article.
References
-
- Emili A. MEC1-dependent phosphorylation of Rad9p in response to DNA damage. Mol. Cell. 1998;2:183–189. - PubMed
-
- Gilbert CS, Green CM, Lowndes NF. Budding yeast Rad9 is an ATP-dependent Rad53 activating machine. Mol. Cell. 2001;8:129–136. - PubMed
-
- Sun Z, Hsiao J, Fay DS, Stern DF. Rad53 FHA domain associated with phosphorylated Rad9 in the DNA damage checkpoint. Science. 1998;281:272–274. - PubMed
Publication types
MeSH terms
Substances
LinkOut - more resources
Full Text Sources
Molecular Biology Databases
Research Materials
Miscellaneous