Structures and mechanisms of viral membrane fusion proteins: multiple variations on a common theme
- PMID: 18568847
- PMCID: PMC2649671
- DOI: 10.1080/10409230802058320
Structures and mechanisms of viral membrane fusion proteins: multiple variations on a common theme
Erratum in
- Crit Rev Biochem Mol Biol. 2008 Jul-Aug;43(4):287-8
Abstract
Recent work has identified three distinct classes of viral membrane fusion proteins based on structural criteria. In addition, there are at least four distinct mechanisms by which viral fusion proteins can be triggered to undergo fusion-inducing conformational changes. Viral fusion proteins also contain different types of fusion peptides and vary in their reliance on accessory proteins. These differing features combine to yield a rich diversity of fusion proteins. Yet despite this staggering diversity, all characterized viral fusion proteins convert from a fusion-competent state (dimers or trimers, depending on the class) to a membrane-embedded homotrimeric prehairpin, and then to a trimer-of-hairpins that brings the fusion peptide, attached to the _target membrane, and the transmembrane domain, attached to the viral membrane, into close proximity thereby facilitating the union of viral and _target membranes. During these conformational conversions, the fusion proteins induce membranes to progress through stages of close apposition, hemifusion, and then the formation of small, and finally large, fusion pores. Clearly, highly divergent proteins have converged on the same overall strategy to mediate fusion, an essential step in the life cycle of every enveloped virus.
Figures
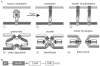
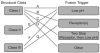
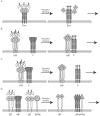
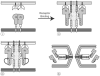
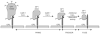
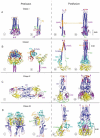
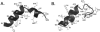
Similar articles
-
A Virion-Based Assay for Glycoprotein Thermostability Reveals Key Determinants of Filovirus Entry and Its Inhibition.J Virol. 2020 Aug 31;94(18):e00336-20. doi: 10.1128/JVI.00336-20. Print 2020 Aug 31. J Virol. 2020. PMID: 32611759 Free PMC article.
-
Influenza Hemifusion Phenotype Depends on Membrane Context: Differences in Cell-Cell and Virus-Cell Fusion.J Mol Biol. 2018 Mar 2;430(5):594-601. doi: 10.1016/j.jmb.2018.01.006. Epub 2018 Feb 2. J Mol Biol. 2018. PMID: 29355500 Free PMC article.
-
Acidic pH-Induced Conformational Changes in Chikungunya Virus Fusion Protein E1: a Spring-Twisted Region in the Domain I-III Linker Acts as a Hinge Point for Swiveling Motion of Domains.J Virol. 2020 Nov 9;94(23):e01561-20. doi: 10.1128/JVI.01561-20. Print 2020 Nov 9. J Virol. 2020. PMID: 32938768 Free PMC article.
-
Viral Membrane Fusion and the Transmembrane Domain.Viruses. 2020 Jun 27;12(7):693. doi: 10.3390/v12070693. Viruses. 2020. PMID: 32604992 Free PMC article. Review.
-
Reovirus FAST proteins: virus-encoded cellular fusogens.Trends Microbiol. 2014 Dec;22(12):715-24. doi: 10.1016/j.tim.2014.08.005. Epub 2014 Sep 19. Trends Microbiol. 2014. PMID: 25245455 Review.
Cited by
-
Identification of the Fusion Peptide-Containing Region in Betacoronavirus Spike Glycoproteins.J Virol. 2016 May 27;90(12):5586-5600. doi: 10.1128/JVI.00015-16. Print 2016 Jun 15. J Virol. 2016. PMID: 27030273 Free PMC article.
-
Envelope glycoprotein of arenaviruses.Viruses. 2012 Oct 17;4(10):2162-81. doi: 10.3390/v4102162. Viruses. 2012. PMID: 23202458 Free PMC article. Review.
-
Single-component multilayered self-assembling nanoparticles presenting rationally designed glycoprotein trimers as Ebola virus vaccines.Nat Commun. 2021 May 11;12(1):2633. doi: 10.1038/s41467-021-22867-w. Nat Commun. 2021. PMID: 33976149 Free PMC article.
-
Assembly of respiratory syncytial virus matrix protein lattice and its coordination with fusion glycoprotein trimers.Nat Commun. 2024 Jul 14;15(1):5923. doi: 10.1038/s41467-024-50162-x. Nat Commun. 2024. PMID: 39004634 Free PMC article.
-
Measles fusion machinery is dysregulated in neuropathogenic variants.mBio. 2015 Feb 10;6(1):e02528-14. doi: 10.1128/mBio.02528-14. mBio. 2015. PMID: 25670774 Free PMC article.
References
-
- Aguilar HC, Matreyek KA, Filone CM, Hashimi ST, Levroney EL, Negrete OA, Bertolotti-Ciarlet A, Choi DY, McHardy I, Fulcher JA, Su SV, Wolf MC, Kohatsu L, Baum LG, Lee B. N-Glycans on Nipah virus fusion protein protect against neutralization but reduce membrane fusion and viral entry. J Virol. 2006;80:4878–4889. - PMC - PubMed
Publication types
MeSH terms
Substances
Grants and funding
LinkOut - more resources
Full Text Sources
Other Literature Sources