Rat prefrontal cortical neurons selectively code strategy switches
- PMID: 19494143
- PMCID: PMC3229282
- DOI: 10.1523/JNEUROSCI.6068-08.2009
Rat prefrontal cortical neurons selectively code strategy switches
Abstract
Multiple memory systems are distinguished by different sets of neuronal circuits and operating principles optimized to solve different problems across mammalian species (Tulving and Schacter, 1994). When a rat selects an arm in a plus maze, for example, the choice can be guided by distinct neural systems (White and Wise, 1999) that encode different relationships among perceived stimuli, actions, and reward. Thus, egocentric or stimulus-response associations require striatal circuits, whereas spatial or episodic learning requires hippocampal circuits (Packard et al., 1989). Although these memory systems function in parallel (Packard and McGaugh, 1996), they can also interact competitively or synergistically (Kim and Ragozzino, 2005). The neuronal mechanisms that coordinate these multiple memory systems are not fully known, but converging evidence suggests that the prefrontal cortex (PFC) is central. The PFC is crucial for abstract, rule-guided behavior in primates and for switching rapidly between memory strategies in rats. We now report that rat medial PFC neuronal activity predicts switching between hippocampus- and caudate-dependent memory strategies. Prelimbic (PL) and infralimbic (IL) neuronal activity changed as rats switched memory strategies even as the rats performed identical behaviors but did not change when rats learned new contingencies using the same strategy. PL dynamics anticipated learning performance whereas IL lagged, suggesting that the two regions help initiate and establish new strategies, respectively. These neuronal dynamics suggest that the PFC contributes to the coordination of memory strategies by integrating the predictive relationships among stimuli, actions, and reward.
Figures
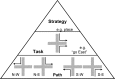
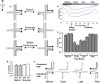
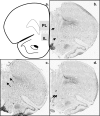
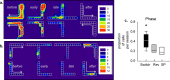
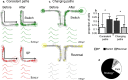
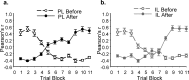
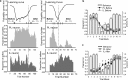
Similar articles
-
Orbitofrontal Cortex Signals Expected Outcomes with Predictive Codes When Stable Contingencies Promote the Integration of Reward History.J Neurosci. 2017 Feb 22;37(8):2010-2021. doi: 10.1523/JNEUROSCI.2951-16.2016. Epub 2017 Jan 23. J Neurosci. 2017. PMID: 28115481 Free PMC article.
-
Prelimbic/infralimbic inactivation impairs memory for multiple task switches, but not flexible selection of familiar tasks.J Neurosci. 2007 Apr 25;27(17):4747-55. doi: 10.1523/JNEUROSCI.0369-07.2007. J Neurosci. 2007. PMID: 17460087 Free PMC article.
-
Dynamic coding of goal-directed paths by orbital prefrontal cortex.J Neurosci. 2011 Apr 20;31(16):5989-6000. doi: 10.1523/JNEUROSCI.5436-10.2011. J Neurosci. 2011. PMID: 21508224 Free PMC article.
-
Parallel processing across neural systems: implications for a multiple memory system hypothesis.Neurobiol Learn Mem. 2004 Nov;82(3):278-98. doi: 10.1016/j.nlm.2004.07.007. Neurobiol Learn Mem. 2004. PMID: 15464410 Review.
-
Involvement of basal ganglia and orbitofrontal cortex in goal-directed behavior.Prog Brain Res. 2000;126:193-215. doi: 10.1016/S0079-6123(00)26015-9. Prog Brain Res. 2000. PMID: 11105648 Review.
Cited by
-
Medial prefrontal cortex predicts internally driven strategy shifts.Neuron. 2015 Apr 8;86(1):331-40. doi: 10.1016/j.neuron.2015.03.015. Epub 2015 Mar 26. Neuron. 2015. PMID: 25819613 Free PMC article.
-
Reversible online control of habitual behavior by optogenetic perturbation of medial prefrontal cortex.Proc Natl Acad Sci U S A. 2012 Nov 13;109(46):18932-7. doi: 10.1073/pnas.1216264109. Epub 2012 Oct 29. Proc Natl Acad Sci U S A. 2012. PMID: 23112197 Free PMC article.
-
Prefrontal deep projection neurons enable cognitive flexibility via persistent feedback monitoring.Cell. 2021 May 13;184(10):2750-2766.e17. doi: 10.1016/j.cell.2021.03.047. Epub 2021 Apr 15. Cell. 2021. PMID: 33861951 Free PMC article.
-
Crowded environments reduce spatial memory in older but not younger adults.Psychol Res. 2018 Mar;82(2):407-428. doi: 10.1007/s00426-016-0819-5. Epub 2016 Oct 25. Psychol Res. 2018. PMID: 27783147
-
Flexible decision-making is related to strategy learning, vicarious trial and error, and medial prefrontal rhythms during spatial set-shifting.Learn Mem. 2024 Jul 22;31(7):a053911. doi: 10.1101/lm.053911.123. Print 2024 Jul. Learn Mem. 2024. PMID: 39038921
References
-
- Asaad WF, Rainer G, Miller EK. Task-specific neural activity in the primate prefrontal cortex. J Neurophysiol. 2000;84:451–459. - PubMed
-
- Brass M, von Cramon DY. Decomposing components of task preparation with functional magnetic resonance imaging. J Cogn Neurosci. 2004;16:609–620. - PubMed
-
- Brown VJ, Bowman EM. Rodent models of prefrontal cortical function. Trends Neurosci. 2002;25:340–343. - PubMed
Publication types
MeSH terms
Grants and funding
LinkOut - more resources
Full Text Sources
Other Literature Sources
Medical
Miscellaneous