Pancreatic cancers require autophagy for tumor growth
- PMID: 21406549
- PMCID: PMC3070934
- DOI: 10.1101/gad.2016111
Pancreatic cancers require autophagy for tumor growth
Abstract
Macroautophagy (autophagy) is a regulated catabolic pathway to degrade cellular organelles and macromolecules. The role of autophagy in cancer is complex and may differ depending on tumor type or context. Here we show that pancreatic cancers have a distinct dependence on autophagy. Pancreatic cancer primary tumors and cell lines show elevated autophagy under basal conditions. Genetic or pharmacologic inhibition of autophagy leads to increased reactive oxygen species, elevated DNA damage, and a metabolic defect leading to decreased mitochondrial oxidative phosphorylation. Together, these ultimately result in significant growth suppression of pancreatic cancer cells in vitro. Most importantly, inhibition of autophagy by genetic means or chloroquine treatment leads to robust tumor regression and prolonged survival in pancreatic cancer xenografts and genetic mouse models. These results suggest that, unlike in other cancers where autophagy inhibition may synergize with chemotherapy or _targeted agents by preventing the up-regulation of autophagy as a reactive survival mechanism, autophagy is actually required for tumorigenic growth of pancreatic cancers de novo, and drugs that inactivate this process may have a unique clinical utility in treating pancreatic cancers and other malignancies with a similar dependence on autophagy. As chloroquine and its derivatives are potent inhibitors of autophagy and have been used safely in human patients for decades for a variety of purposes, these results are immediately translatable to the treatment of pancreatic cancer patients, and provide a much needed, novel vantage point of attack.
Figures
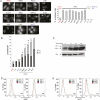
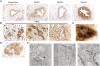
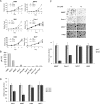
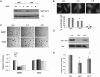
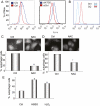
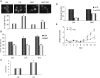
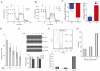
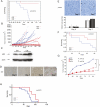
Similar articles
-
A critical role for autophagy in pancreatic cancer.Autophagy. 2011 Aug;7(8):912-3. doi: 10.4161/auto.7.8.15762. Epub 2011 Aug 1. Autophagy. 2011. PMID: 21494085
-
Chloroquine _targets pancreatic cancer stem cells via inhibition of CXCR4 and hedgehog signaling.Mol Cancer Ther. 2014 Jul;13(7):1758-71. doi: 10.1158/1535-7163.MCT-13-0948. Epub 2014 Apr 30. Mol Cancer Ther. 2014. PMID: 24785258
-
NLRP4 renders pancreatic cancer resistant to olaparib through promotion of the DNA damage response and ROS-induced autophagy.Cell Death Dis. 2024 Aug 26;15(8):620. doi: 10.1038/s41419-024-06984-0. Cell Death Dis. 2024. PMID: 39187531 Free PMC article.
-
Autophagy as a therapeutic _target in pancreatic cancer.Br J Cancer. 2021 Jan;124(2):333-344. doi: 10.1038/s41416-020-01039-5. Epub 2020 Sep 15. Br J Cancer. 2021. PMID: 32929194 Free PMC article. Review.
-
Autophagy Inhibition in Pancreatic Adenocarcinoma.Clin Colorectal Cancer. 2018 Mar;17(1):25-31. doi: 10.1016/j.clcc.2017.10.013. Epub 2017 Oct 28. Clin Colorectal Cancer. 2018. PMID: 29223362 Review.
Cited by
-
Role of autophagy in the resistance to tumour necrosis factor-related apoptosis-inducing ligand-induced apoptosis in papillary and anaplastic thyroid cancer cells.Endocrine. 2014 Mar;45(2):256-62. doi: 10.1007/s12020-013-9997-8. Epub 2013 Jul 3. Endocrine. 2014. PMID: 23821365
-
Mutant KRAS drives metabolic reprogramming and autophagic flux in premalignant pancreatic cells.Cancer Gene Ther. 2022 May;29(5):505-518. doi: 10.1038/s41417-021-00326-4. Epub 2021 Apr 8. Cancer Gene Ther. 2022. PMID: 33833413 Free PMC article.
-
HDAC Family Members Intertwined in the Regulation of Autophagy: A Druggable Vulnerability in Aggressive Tumor Entities.Cells. 2015 Apr 23;4(2):135-68. doi: 10.3390/cells4020135. Cells. 2015. PMID: 25915736 Free PMC article. Review.
-
Interplays of glucose metabolism and KRAS mutation in pancreatic ductal adenocarcinoma.Cell Death Dis. 2022 Sep 24;13(9):817. doi: 10.1038/s41419-022-05259-w. Cell Death Dis. 2022. PMID: 36151074 Free PMC article. Review.
-
Human pancreatic cancer tumors are nutrient poor and tumor cells actively scavenge extracellular protein.Cancer Res. 2015 Feb 1;75(3):544-53. doi: 10.1158/0008-5472.CAN-14-2211. Cancer Res. 2015. PMID: 25644265 Free PMC article.
References
-
- Bellodi C, Lidonnici MR, Hamilton A, Helgason GV, Soliera AR, Ronchetti M, Galavotti S, Young KW, Selmi T, Yacobi R, et al. 2009. _targeting autophagy potentiates tyrosine kinase inhibitor-induced cell death in Philadelphia chromosome-positive cells, including primary CML stem cells. J Clin Invest 119: 1109–1123 - PMC - PubMed
-
- Ben-Josef E, Lawrence TS 2008. Chemoradiotherapy for unresectable pancreatic cancer. Int J Clin Oncol 13: 121–126 - PubMed
-
- Bjorkoy G, Lamark T, Pankiv S, Øvervatn A, Brech A, Johansen T 2009. Monitoring autophagic degradation of p62/SQSTM1. Methods Enzymol 452: 181–197 - PubMed
Publication types
MeSH terms
Substances
Grants and funding
LinkOut - more resources
Full Text Sources
Other Literature Sources
Medical
Research Materials