Tissue organization by cadherin adhesion molecules: dynamic molecular and cellular mechanisms of morphogenetic regulation
- PMID: 21527735
- PMCID: PMC3556819
- DOI: 10.1152/physrev.00004.2010
Tissue organization by cadherin adhesion molecules: dynamic molecular and cellular mechanisms of morphogenetic regulation
Abstract
This review addresses the cellular and molecular mechanisms of cadherin-based tissue morphogenesis. Tissue physiology is profoundly influenced by the distinctive organizations of cells in organs and tissues. In metazoa, adhesion receptors of the classical cadherin family play important roles in establishing and maintaining such tissue organization. Indeed, it is apparent that cadherins participate in a range of morphogenetic events that range from support of tissue integrity to dynamic cellular rearrangements. A comprehensive understanding of cadherin-based morphogenesis must then define the molecular and cellular mechanisms that support these distinct cadherin biologies. Here we focus on four key mechanistic elements: the molecular basis for adhesion through cadherin ectodomains, the regulation of cadherin expression at the cell surface, cooperation between cadherins and the actin cytoskeleton, and regulation by cell signaling. We discuss current progress and outline issues for further research in these fields.
Figures
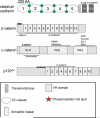
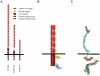
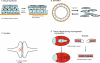
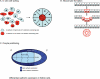
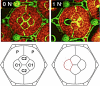
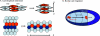
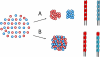
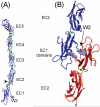
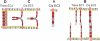
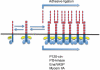
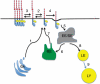
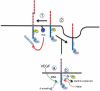
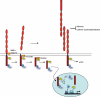
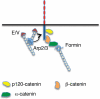
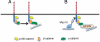
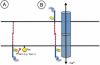
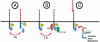
Similar articles
-
Cadherin cell adhesion receptors as a morphogenetic regulator.Science. 1991 Mar 22;251(5000):1451-5. doi: 10.1126/science.2006419. Science. 1991. PMID: 2006419 Review.
-
Cadherins in development: cell adhesion, sorting, and tissue morphogenesis.Genes Dev. 2006 Dec 1;20(23):3199-214. doi: 10.1101/gad.1486806. Genes Dev. 2006. PMID: 17158740 Review.
-
Classical cadherin adhesion molecules: coordinating cell adhesion, signaling and the cytoskeleton.J Mol Histol. 2004 Nov;35(8-9):839-44. doi: 10.1007/s10735-004-1833-2. J Mol Histol. 2004. PMID: 15609097 Review.
-
Cell adhesion: the molecular basis of tissue architecture and morphogenesis.Cell. 1996 Feb 9;84(3):345-57. doi: 10.1016/s0092-8674(00)81279-9. Cell. 1996. PMID: 8608588 Review.
-
Cadherin-catenin complex: protein interactions and their implications for cadherin function.J Cell Biochem. 1996 Jun 15;61(4):514-23. doi: 10.1002/(SICI)1097-4644(19960616)61:4%3C514::AID-JCB4%3E3.0.CO;2-R. J Cell Biochem. 1996. PMID: 8806074 Review.
Cited by
-
Activation of cell-surface proteases promotes necroptosis, inflammation and cell migration.Cell Res. 2016 Aug;26(8):886-900. doi: 10.1038/cr.2016.87. Epub 2016 Jul 22. Cell Res. 2016. PMID: 27444869 Free PMC article.
-
Cooperation of distinct Rac-dependent pathways to stabilise E-cadherin adhesion.Cell Signal. 2015 Sep;27(9):1905-13. doi: 10.1016/j.cellsig.2015.04.014. Epub 2015 May 5. Cell Signal. 2015. PMID: 25957131 Free PMC article.
-
Thermo-chemotherapy Induced miR-218 upregulation inhibits the invasion of gastric cancer via _targeting Gli2 and E-cadherin.Tumour Biol. 2015 Aug;36(8):5807-14. doi: 10.1007/s13277-015-3250-4. Epub 2015 Feb 19. Tumour Biol. 2015. PMID: 25694126
-
Cadherin complexity: recent insights into cadherin superfamily function in C. elegans.Curr Opin Cell Biol. 2012 Oct;24(5):695-701. doi: 10.1016/j.ceb.2012.06.008. Epub 2012 Jul 19. Curr Opin Cell Biol. 2012. PMID: 22819515 Free PMC article. Review.
-
Mesenchymal Stem Cells in the Adult Human Liver: Hype or Hope?Cells. 2019 Sep 22;8(10):1127. doi: 10.3390/cells8101127. Cells. 2019. PMID: 31546729 Free PMC article. Review.
References
-
- Abe K, Chisaka O, van Roy F, Takeichi M. Stability of dendritic spines and synaptic contacts is controlled by aN-catenin. Nat Neurosci. 2004 - PubMed
-
- Akhmanova A, Stehbens SJ, Yap AS. Touch, grasp, deliver and control: functional cross-talk between microtubules and cell adhesions. Traffic. 2009;10:268–274. - PubMed
Publication types
MeSH terms
Substances
Grants and funding
LinkOut - more resources
Full Text Sources
Other Literature Sources