Expression and secretion of TNF-α in mouse taste buds: a novel function of a specific subset of type II taste cells
- PMID: 22905218
- PMCID: PMC3419207
- DOI: 10.1371/journal.pone.0043140
Expression and secretion of TNF-α in mouse taste buds: a novel function of a specific subset of type II taste cells
Abstract
Taste buds are chemosensory structures widely distributed on the surface of the oral cavity and larynx. Taste cells, exposed to the oral environment, face great challenges in defense against potential pathogens. While immune cells, such as T-cells and macrophages, are rarely found in taste buds, high levels of expression of some immune-response-associated molecules are observed in taste buds. Yet, the cellular origins of these immune molecules such as cytokines in taste buds remain to be determined. Here, we show that a specific subset of taste cells selectively expresses high levels of the inflammatory cytokine tumor necrosis factor-α (TNF-α). Based on immuno-colocalization experiments using taste-cell-type markers, the TNF-α-producing cells are predominantly type II taste cells expressing the taste receptor T1R3. These cells can rapidly increase TNF-α production and secretion upon inflammatory challenges, both in vivo and in vitro. The lipopolysaccharide (LPS)-induced TNF-α expression in taste cells was completely eliminated in TLR2(-/-)/TLR4(-/-) double-gene-knockout mice, which confirms that the induction of TNF-α in taste buds by LPS is mediated through TLR signaling pathways. The taste-cell-produced TNF-α may contribute to local immune surveillance, as well as regulate taste sensation under normal and pathological conditions.
Conflict of interest statement
Figures
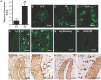
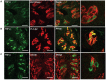
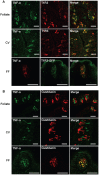
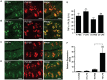
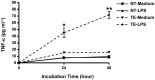
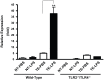
Similar articles
-
Interleukin-10 is produced by a specific subset of taste receptor cells and critical for maintaining structural integrity of mouse taste buds.J Neurosci. 2014 Feb 12;34(7):2689-701. doi: 10.1523/JNEUROSCI.3074-13.2014. J Neurosci. 2014. PMID: 24523558 Free PMC article.
-
Activation of Toll-like receptors by Burkholderia pseudomallei.BMC Immunol. 2008 Aug 8;9:46. doi: 10.1186/1471-2172-9-46. BMC Immunol. 2008. PMID: 18691413 Free PMC article.
-
Effects of budesonide on toll-like receptor expression in alveolar macrophages from smokers with and without COPD.Int J Chron Obstruct Pulmon Dis. 2016 May 17;11:1035-43. doi: 10.2147/COPD.S102668. eCollection 2016. Int J Chron Obstruct Pulmon Dis. 2016. PMID: 27274225 Free PMC article.
-
Inhibition of lipopolysaccharide-induced signal transduction in endotoxin-tolerized mouse macrophages: dysregulation of cytokine, chemokine, and toll-like receptor 2 and 4 gene expression.J Immunol. 2000 Jun 1;164(11):5564-74. doi: 10.4049/jimmunol.164.11.5564. J Immunol. 2000. PMID: 10820230
-
Regulation of bitter taste responses by tumor necrosis factor.Brain Behav Immun. 2015 Oct;49:32-42. doi: 10.1016/j.bbi.2015.04.001. Epub 2015 Apr 21. Brain Behav Immun. 2015. PMID: 25911043 Free PMC article.
Cited by
-
Tuft Cells: Context- and Tissue-Specific Programming for a Conserved Cell Lineage.Annu Rev Pathol. 2023 Jan 24;18:311-335. doi: 10.1146/annurev-pathol-042320-112212. Epub 2022 Nov 9. Annu Rev Pathol. 2023. PMID: 36351364 Free PMC article. Review.
-
Tongue immune compartment analysis reveals spatial macrophage heterogeneity.Elife. 2022 Jun 24;11:e77490. doi: 10.7554/eLife.77490. Elife. 2022. PMID: 35749158 Free PMC article.
-
Adaptive immune cells shape obesity-associated type 2 diabetes mellitus and less prominent comorbidities.Nat Rev Endocrinol. 2022 Jan;18(1):23-42. doi: 10.1038/s41574-021-00575-1. Epub 2021 Oct 26. Nat Rev Endocrinol. 2022. PMID: 34703027 Free PMC article. Review.
-
Whole transcriptome profiling of taste bud cells.Sci Rep. 2017 Aug 8;7(1):7595. doi: 10.1038/s41598-017-07746-z. Sci Rep. 2017. PMID: 28790351 Free PMC article.
-
Physiology of the tongue with emphasis on taste transduction.Physiol Rev. 2023 Apr 1;103(2):1193-1246. doi: 10.1152/physrev.00012.2022. Epub 2022 Nov 24. Physiol Rev. 2023. PMID: 36422992 Free PMC article. Review.
References
Publication types
MeSH terms
Substances
Grants and funding
LinkOut - more resources
Full Text Sources
Molecular Biology Databases