Vitronectin induces phosphorylation of ezrin/radixin/moesin actin-binding proteins through binding to its novel neuronal receptor telencephalin
- PMID: 23019340
- PMCID: PMC3493945
- DOI: 10.1074/jbc.M112.383851
Vitronectin induces phosphorylation of ezrin/radixin/moesin actin-binding proteins through binding to its novel neuronal receptor telencephalin
Abstract
Vitronectin (VN) is an extracellular matrix protein abundantly present in blood and a wide variety of tissues and plays important roles in a number of biological phenomena mainly through its binding to αV integrins. However, its definite function in the brain remains largely unknown. Here we report the identification of telencephalin (TLCN/ICAM-5) as a novel VN receptor on neuronal dendrites. VN strongly binds to TLCN, a unique neuronal member of the ICAM family, which is specifically expressed on dendrites of spiny neurons in the mammalian telencephalon. VN-coated microbeads induce the formation of phagocytic cup-like plasma membrane protrusions on dendrites of cultured hippocampal neurons and trigger the activation of TLCN-dependent intracellular signaling cascade including the phosphorylation of ezrin/radixin/moesin actin-binding proteins and recruitment of F-actin and phosphatidylinositol 4,5-bisphosphate for morphological transformation of the dendritic protrusions. These results suggest that the extracellular matrix molecule VN and its neuronal receptor TLCN play a pivotal role in the phosphorylation of ezrin/radixin/moesin proteins and the formation of phagocytic cup-like structures on neuronal dendrites.
Figures
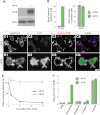
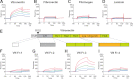
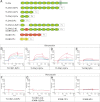
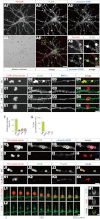
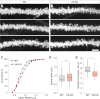
Similar articles
-
Purification of the Dendritic Filopodia-rich Fraction.J Vis Exp. 2019 May 2;(147). doi: 10.3791/59292. J Vis Exp. 2019. PMID: 31107457
-
Interaction between telencephalin and ERM family proteins mediates dendritic filopodia formation.J Neurosci. 2007 Aug 15;27(33):8866-76. doi: 10.1523/JNEUROSCI.1047-07.2007. J Neurosci. 2007. PMID: 17699668 Free PMC article.
-
A novel phenylalanine-based _targeting signal directs telencephalin to neuronal dendrites.J Neurosci. 2005 Feb 2;25(5):1122-31. doi: 10.1523/JNEUROSCI.3853-04.2005. J Neurosci. 2005. PMID: 15689548 Free PMC article.
-
Regulation of cortical structure by the ezrin-radixin-moesin protein family.Curr Opin Cell Biol. 1999 Feb;11(1):109-16. doi: 10.1016/s0955-0674(99)80013-1. Curr Opin Cell Biol. 1999. PMID: 10047517 Review.
-
Two Sides of the Coin: Ezrin/Radixin/Moesin and Merlin Control Membrane Structure and Contact Inhibition.Int J Mol Sci. 2019 Apr 23;20(8):1996. doi: 10.3390/ijms20081996. Int J Mol Sci. 2019. PMID: 31018575 Free PMC article. Review.
Cited by
-
ICAM-5 affects spine maturation by regulation of NMDA receptor binding to α-actinin.Biol Open. 2015 Jan 8;4(2):125-36. doi: 10.1242/bio.201410439. Biol Open. 2015. PMID: 25572420 Free PMC article.
-
Proteomic Analysis of Dendritic Filopodia-Rich Fraction Isolated by Telencephalin and Vitronectin Interaction.Front Synaptic Neurosci. 2018 Aug 10;10:27. doi: 10.3389/fnsyn.2018.00027. eCollection 2018. Front Synaptic Neurosci. 2018. PMID: 30147651 Free PMC article.
-
Comparative- and network-based proteomic analysis of bacterial chondronecrosis with osteomyelitis lesions in broiler's proximal tibiae identifies new molecular signatures of lameness.Sci Rep. 2023 Apr 12;13(1):5947. doi: 10.1038/s41598-023-33060-y. Sci Rep. 2023. PMID: 37045932 Free PMC article.
-
Monoallelic loss of the F-actin-binding protein radixin facilitates startle reactivity and pre-pulse inhibition in mice.Front Cell Dev Biol. 2022 Nov 28;10:987691. doi: 10.3389/fcell.2022.987691. eCollection 2022. Front Cell Dev Biol. 2022. PMID: 36518539 Free PMC article.
-
Urokinase-type plasminogen activator (uPA) promotes ezrin-mediated reorganization of the synaptic cytoskeleton in the ischemic brain.J Biol Chem. 2018 Jun 15;293(24):9234-9247. doi: 10.1074/jbc.RA118.002534. Epub 2018 May 2. J Biol Chem. 2018. PMID: 29720403 Free PMC article.
References
-
- Geiger B., Bershadsky A., Pankov R., Yamada K. M. (2001) Transmembrane cross-talk between the extracellular matrix-cytoskeleton cross-talk. Nat. Rev. Mol. Cell Biol. 2, 793–805 - PubMed
-
- Dityatev A., Schachner M., Sonderegger P. (2010) The dual role of the extracellular matrix in synaptic plasticity and homeostasis. Nat. Rev. Neurosci. 11, 735–746 - PubMed
-
- Preissner K. T. (1991) Structure and biological role of vitronectin. Annu. Rev. Cell Biol. 7, 275–310 - PubMed
-
- Niquet J., Gillian A., Ben-Ari Y., Represa A. (1996) Reactive glial cells express a vitronectin-like protein in the hippocampus of epileptic rats. Glia 16, 359–367 - PubMed
-
- Seiffert D., Iruela-Arispe M. L., Sage E. H., Loskutoff D. J. (1995) Distribution of vitronectin mRNA during murine development. Dev. Dyn. 203, 71–79 - PubMed
Publication types
MeSH terms
Substances
LinkOut - more resources
Full Text Sources
Molecular Biology Databases
Research Materials