Regulation of nutrient-sensitive autophagy by uncoordinated 51-like kinases 1 and 2
- PMID: 23291478
- PMCID: PMC3590256
- DOI: 10.4161/auto.23066
Regulation of nutrient-sensitive autophagy by uncoordinated 51-like kinases 1 and 2
Abstract
Macroautophagy, commonly referred to as autophagy, is a protein degradation pathway that occurs constitutively in cells, but can also be induced by stressors such as nutrient starvation or protein aggregation. Autophagy has been implicated in multiple disease mechanisms including neurodegeneration and cancer, with both tumor suppressive and oncogenic roles. Uncoordinated 51-like kinase 1 (ULK1) is a critical autophagy protein near the apex of the hierarchal regulatory pathway that receives signals from the master nutrient sensors MTOR and AMP-activated protein kinase (AMPK). In mammals, ULK1 has a close homolog, ULK2, although their functional distinctions have been unclear. Here, we show that ULK1 and ULK2 both function to support autophagy activation following nutrient starvation. Increased autophagy following amino acid or glucose starvation was disrupted only upon combined loss of ULK1 and ULK2 in mouse embryonic fibroblasts. Generation of PtdIns3P and recruitment of WIPI2 or ZFYVE1/DFCP1 to the phagophore following amino acid starvation was blocked by combined Ulk1/2 double knockout. Autophagy activation following glucose starvation did not involve recruitment of either WIPI1 or WIPI2 to forming autophagosomes. Consistent with a PtdIns3P-independent mechanism, glucose-dependent autophagy was resistant to wortmannin. Our findings support functional redundancy between ULK1 and ULK2 for nutrient-dependent activation of autophagy and furthermore highlight the differential pathways that respond to amino acid and glucose deprivation.
Keywords: MEF; ULK1; ULK2; WIPI1; WIPI2; knockout; nutrient starvation.
Figures
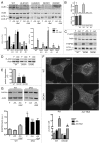
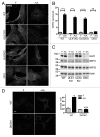
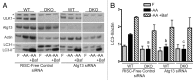
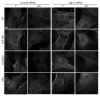
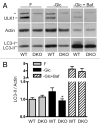
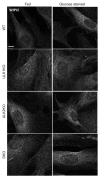
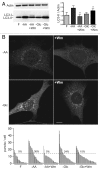
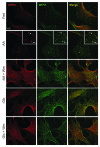
Similar articles
-
AMPK Inhibits ULK1-Dependent Autophagosome Formation and Lysosomal Acidification via Distinct Mechanisms.Mol Cell Biol. 2018 Apr 30;38(10):e00023-18. doi: 10.1128/MCB.00023-18. Print 2018 May 15. Mol Cell Biol. 2018. PMID: 29507183 Free PMC article.
-
Ammonia-induced autophagy is independent of ULK1/ULK2 kinases.Proc Natl Acad Sci U S A. 2011 Jul 5;108(27):11121-6. doi: 10.1073/pnas.1107969108. Epub 2011 Jun 20. Proc Natl Acad Sci U S A. 2011. PMID: 21690395 Free PMC article.
-
Nutrient starvation elicits an acute autophagic response mediated by Ulk1 dephosphorylation and its subsequent dissociation from AMPK.Proc Natl Acad Sci U S A. 2011 Mar 22;108(12):4788-93. doi: 10.1073/pnas.1100844108. Epub 2011 Mar 7. Proc Natl Acad Sci U S A. 2011. PMID: 21383122 Free PMC article.
-
So Many Roads: the Multifaceted Regulation of Autophagy Induction.Mol Cell Biol. 2018 Oct 15;38(21):e00303-18. doi: 10.1128/MCB.00303-18. Print 2018 Nov 1. Mol Cell Biol. 2018. PMID: 30126896 Free PMC article. Review.
-
Role of an intrinsically disordered conformation in AMPK-mediated phosphorylation of ULK1 and regulation of autophagy.Mol Biosyst. 2012 Jan;8(1):91-6. doi: 10.1039/c1mb05265a. Epub 2011 Aug 18. Mol Biosyst. 2012. PMID: 21853163 Review.
Cited by
-
Recruitment and Activation of the ULK1/Atg1 Kinase Complex in Selective Autophagy.J Mol Biol. 2020 Jan 3;432(1):123-134. doi: 10.1016/j.jmb.2019.07.027. Epub 2019 Jul 25. J Mol Biol. 2020. PMID: 31351898 Free PMC article. Review.
-
Specific microRNAs for Modulation of Autophagy in Spinal Cord Injury.Brain Sci. 2022 Feb 11;12(2):247. doi: 10.3390/brainsci12020247. Brain Sci. 2022. PMID: 35204010 Free PMC article. Review.
-
Interplay of energy metabolism and autophagy.Autophagy. 2024 Jan;20(1):4-14. doi: 10.1080/15548627.2023.2247300. Epub 2023 Aug 18. Autophagy. 2024. PMID: 37594406 Free PMC article. Review.
-
Structure and function of the ULK1 complex in autophagy.Curr Opin Cell Biol. 2016 Apr;39:61-8. doi: 10.1016/j.ceb.2016.02.010. Epub 2016 Feb 27. Curr Opin Cell Biol. 2016. PMID: 26921696 Free PMC article. Review.
-
Mirror image phosphoinositides regulate autophagy.Mol Cell Oncol. 2015 Feb 26;3(2):e1019974. doi: 10.1080/23723556.2015.1019974. eCollection 2016 Mar. Mol Cell Oncol. 2015. PMID: 27308581 Free PMC article.
References
Publication types
MeSH terms
Substances
Grants and funding
LinkOut - more resources
Full Text Sources
Other Literature Sources
Molecular Biology Databases
Research Materials
Miscellaneous