Multiple mechanisms of extracellular tau spreading in a non-transgenic tauopathy model
- PMID: 23383401
- PMCID: PMC3560471
Multiple mechanisms of extracellular tau spreading in a non-transgenic tauopathy model
Abstract
While the interneuronal propagation of neurofibrillary lesions in Alzheimer's disease and other tauopathies now appears to involve the spreading of tau-associated toxicity, little is known about its mechanism. We characterized the movement of human tau through the brain of a non-transgenic lower vertebrate tauopathy model in which full-length wild type and mutant human tau isoforms were expressed in identified neurons, thus permitting the identification and localization of EC tau sources. We describe two distinct patterns of tau spreading that correspond to tau species that lack (MTBR-) and contain (MTBR+) the tau microtubule-binding region. These patterns illustrate the production, migration and uptake of EC tau and resemble some of the extracellular tau deposits typically seen in human brain after repeated traumatic injury in cases of chronic traumatic encephalopathy (CTE). We propose that misprocessed human tau can spread between CNS neurons via a variety of non-synaptic mechanisms as well as synaptically mediated mechanisms.
Keywords: CSF-tau; chronic traumatic encephalopathy; interneuronal lesion spread; neuron death; tau secretion.
Figures
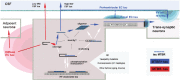
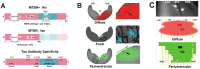
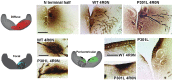
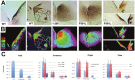
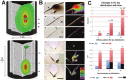
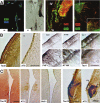
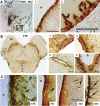
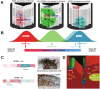
Similar articles
-
Interneuronal transfer of human tau between Lamprey central neurons in situ.J Alzheimers Dis. 2010;19(2):647-64. doi: 10.3233/JAD-2010-1273. J Alzheimers Dis. 2010. PMID: 20110609
-
Anterograde and Retrograde Propagation of Inoculated Human Tau Fibrils and Tau Oligomers in a Non-Transgenic Rat Tauopathy Model.Biomedicines. 2023 Mar 24;11(4):1004. doi: 10.3390/biomedicines11041004. Biomedicines. 2023. PMID: 37189622 Free PMC article.
-
Evidence for accelerated tauopathy in the retina of transgenic P301S tau mice exposed to repetitive mild traumatic brain injury.Exp Neurol. 2015 Nov;273:168-76. doi: 10.1016/j.expneurol.2015.08.014. Epub 2015 Aug 24. Exp Neurol. 2015. PMID: 26311071
-
The role of extracellular Tau in the spreading of neurofibrillary pathology.Front Cell Neurosci. 2014 Apr 23;8:113. doi: 10.3389/fncel.2014.00113. eCollection 2014. Front Cell Neurosci. 2014. PMID: 24795568 Free PMC article. Review.
-
Traumatic brain injury (TBI) in collision sports: Possible mechanisms of transformation into chronic traumatic encephalopathy (CTE).Metabolism. 2019 Nov;100S:153943. doi: 10.1016/j.metabol.2019.07.007. Metabolism. 2019. PMID: 31610856 Review.
Cited by
-
Extracellular Membrane Vesicles as Vehicles for Brain Cell-to-Cell Interactions in Physiological as well as Pathological Conditions.Biomed Res Int. 2015;2015:152926. doi: 10.1155/2015/152926. Epub 2015 Oct 25. Biomed Res Int. 2015. PMID: 26583089 Free PMC article. Review.
-
Recent Preclinical Insights Into the Treatment of Chronic Traumatic Encephalopathy.Front Neurosci. 2020 Jul 7;14:616. doi: 10.3389/fnins.2020.00616. eCollection 2020. Front Neurosci. 2020. PMID: 32774238 Free PMC article. Review.
-
Proteins recruited to exosomes by tau overexpression implicate novel cellular mechanisms linking tau secretion with Alzheimer's disease.J Alzheimers Dis. 2014;40 Suppl 1(Suppl 1):S47-70. doi: 10.3233/JAD-132135. J Alzheimers Dis. 2014. PMID: 24718102 Free PMC article.
-
The Distance between N and C Termini of Tau and of FTDP-17 Mutants Is Modulated by Microtubule Interactions in Living Cells.Front Mol Neurosci. 2017 Jun 30;10:210. doi: 10.3389/fnmol.2017.00210. eCollection 2017. Front Mol Neurosci. 2017. PMID: 28713242 Free PMC article.
-
Could α-synuclein amyloid-like aggregates trigger a prionic neuronal invasion?Biomed Res Int. 2015;2015:172018. doi: 10.1155/2015/172018. Epub 2015 Mar 19. Biomed Res Int. 2015. PMID: 25866763 Free PMC article. Review.
References
-
- Spillantini MG, Goedert M. Tau protein pathology in neurodegenerative diseases. Trends Neurosci. 1998;10:428–433. - PubMed
-
- Lee VM, Goedert M, Trojanowski JQ. Neurodegenerative tauopathies. Annu Rev Neurosci. 2001;24:1121–1159. - PubMed
-
- Avila J. Tau phosphorylation and aggregation in Alzheimer's disease pathology. FEBS Lett. 2006;580:2922–2927. - PubMed
-
- Stoothoff WH, Johnson GV. Tau phosphorylation: physiological and pathological consequences. Biochim Biophys Acta. 2005;1739:280–297. - PubMed
-
- Braak H, Braak E. Neuropathological staging of Alzheimer-related changes. Acta Neuropath. 1991;82:239–259. - PubMed
LinkOut - more resources
Full Text Sources
Other Literature Sources