Defective counterregulation and hypoglycemia unawareness in diabetes: mechanisms and emerging treatments
- PMID: 23391237
- PMCID: PMC3568263
- DOI: 10.1016/j.ecl.2012.11.005
Defective counterregulation and hypoglycemia unawareness in diabetes: mechanisms and emerging treatments
Abstract
For people with diabetes, hypoglycemia remains the limiting factor in achieving glycemic control. This article reviews recent advances in how the brain senses and responds to hypoglycemia. Novel mechanisms by which individuals with insulin-treated diabetes develop hypoglycemia unawareness and impaired counterregulatory responses are outlined. Prevention strategies for reducing the incidence of hypoglycemia are discussed.
Copyright © 2013 Elsevier Inc. All rights reserved.
Figures
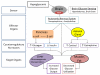
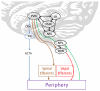
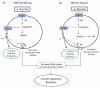
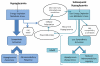
Similar articles
-
Hypoglycemia in diabetes.Diabetes Care. 2003 Jun;26(6):1902-12. doi: 10.2337/diacare.26.6.1902. Diabetes Care. 2003. PMID: 12766131 Review.
-
Mechanisms of hypoglycemia-associated autonomic failure and its component syndromes in diabetes.Diabetes. 2005 Dec;54(12):3592-601. doi: 10.2337/diabetes.54.12.3592. Diabetes. 2005. PMID: 16306382 Review.
-
Hypoglycemia-associated autonomic failure in diabetes.Am J Physiol Endocrinol Metab. 2001 Dec;281(6):E1115-21. doi: 10.1152/ajpendo.2001.281.6.E1115. Am J Physiol Endocrinol Metab. 2001. PMID: 11701423 Review.
-
Hypoglycemia in diabetes: pathophysiological mechanisms and diurnal variation.Prog Brain Res. 2006;153:361-5. doi: 10.1016/S0079-6123(06)53021-3. Prog Brain Res. 2006. PMID: 16876586 Review.
-
Hypoglycemia unawareness.Diabetes Res Clin Pract. 2011 Aug;93 Suppl 1:S92-6. doi: 10.1016/S0168-8227(11)70020-1. Diabetes Res Clin Pract. 2011. PMID: 21864759 Review.
Cited by
-
A Systematic Review of Neuroprotective Strategies in the Management of Hypoglycemia.Int J Mol Sci. 2019 Jan 28;20(3):550. doi: 10.3390/ijms20030550. Int J Mol Sci. 2019. PMID: 30696060 Free PMC article.
-
Ghrelin does not impact the blunted counterregulatory response to recurrent hypoglycemia in mice.Front Endocrinol (Lausanne). 2023 Jun 2;14:1181856. doi: 10.3389/fendo.2023.1181856. eCollection 2023. Front Endocrinol (Lausanne). 2023. PMID: 37334290 Free PMC article.
-
Hypoglycemia induced by insulin as a triggering factor of cognitive deficit in diabetic children.ScientificWorldJournal. 2014 Mar 23;2014:616534. doi: 10.1155/2014/616534. eCollection 2014. ScientificWorldJournal. 2014. PMID: 24790575 Free PMC article. Review.
-
Standardizing Clinically Meaningful Outcome Measures Beyond HbA1c for Type 1 Diabetes: A Consensus Report of the American Association of Clinical Endocrinologists, the American Association of Diabetes Educators, the American Diabetes Association, the Endocrine Society, JDRF International, The Leona M. and Harry B. Helmsley Charitable Trust, the Pediatric Endocrine Society, and the T1D Exchange.Diabetes Care. 2017 Dec;40(12):1622-1630. doi: 10.2337/dc17-1624. Diabetes Care. 2017. PMID: 29162582 Free PMC article. Review.
-
Recurrent hypoglycemia: boosting the brain's metabolic flexibility.J Clin Invest. 2013 May;123(5):1922-4. doi: 10.1172/JCI69796. Epub 2013 Apr 1. J Clin Invest. 2013. PMID: 23543052 Free PMC article.
References
-
- Duckworth W, Abraira C, Moritz T, et al. Glucose Control and Vascular Complications in Veterans with Type 2 Diabetes. New England Journal of Medicine. 2009;360:129–U162. - PubMed
-
- Terry T, Raravikar K, Chokrungvaranon N, et al. Does aggressive glycemic control benefit macrovascular and microvascular disease in type 2 diabetes? Insights from ACCORD, ADVANCE, and VADT. Curr Cardiol Rep. 2012;14:79–88. - PubMed
-
- The Diabetes Control and Complications Trial Research Group The effect of intensive treatment of diabetes on the development and progression of lon-term complications in insulin-dependent diabetes mellitus. New England Journal of Medicine. 1993;329:977–996. - PubMed
-
- Turner RC, Holman RR, Stratton IM, et al. Effect of intensive blood-glucose control with metformin on complications in overweight patients with type 2 diabetes (UKPDS 34) Lancet. 1998;352:854–865. - PubMed
-
- The ADVANCE collaborative group Intensive blood glucose control and vascular outcomes in patients with type 2 diabetes. New England Journal of Medicine. 2008:358. - PubMed
Publication types
MeSH terms
Substances
Grants and funding
LinkOut - more resources
Full Text Sources
Other Literature Sources
Medical