PTB-associated splicing factor (PSF) is a PPARγ-binding protein and growth regulator of colon cancer cells
- PMID: 23516550
- PMCID: PMC3596311
- DOI: 10.1371/journal.pone.0058749
PTB-associated splicing factor (PSF) is a PPARγ-binding protein and growth regulator of colon cancer cells
Abstract
Peroxisome proliferator-activated receptor gamma (PPARγ) is a nuclear receptor that plays an essential role in cell proliferation, apoptosis, and inflammation. It is over-expressed in many types of cancer, including colon, stomach, breast, and lung cancer, suggesting that regulation of PPARγ might affect cancer pathogenesis. Here, using a proteomic approach, we identify PTB-associated splicing factor (PSF) as a novel PPARγ-interacting protein and demonstrate that PSF is involved in several important regulatory steps of colon cancer cell proliferation. To investigate the relationship between PSF and PPARγ in colon cancer, we evaluated the effects of PSF expression in DLD-1 and HT-29 colon cancer cell lines, which express low and high levels of PPARγ, respectively PSF affected the ability of PPARγ to bind, and expression of PSF siRNA significantly suppressed the proliferation of colon cancer cells. Furthermore, PSF knockdown induced apoptosis via activation of caspase-3. Interestingly, DLD-1 cells were more susceptible to PSF knockdown-induced cell death than HT-29 cells. Our data suggest that PSF is an important regulator of cell death that plays critical roles in the survival and growth of colon cancer cells. The PSF-PPARγ axis may play a role in the control of colorectal carcinogenesis. Taken together, this study is the first to describe the effects of PSF on cell proliferation, tumor growth, and cell signaling associated with PPARγ.
Conflict of interest statement
Figures
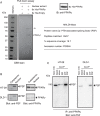
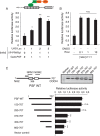
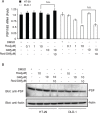
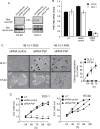
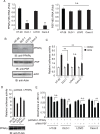
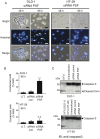
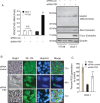
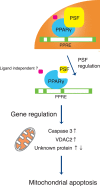
Similar articles
-
PSF knockdown enhances apoptosis via downregulation of LC3B in human colon cancer cells.Biomed Res Int. 2013;2013:204973. doi: 10.1155/2013/204973. Epub 2013 Oct 31. Biomed Res Int. 2013. PMID: 24288667 Free PMC article.
-
The PTB-Associated Splicing Factor/Peroxisome Proliferator-Activated Receptor Gamma Axis Regulates Autophagosome Formation in Human Pancreatic Cancer Cells.Biores Open Access. 2015 Jul 1;4(1):319-25. doi: 10.1089/biores.2015.0018. eCollection 2015. Biores Open Access. 2015. PMID: 26309807 Free PMC article.
-
Peroxisome proliferator-activated receptor gamma overexpression suppresses proliferation of human colon cancer cells.Biochem Biophys Res Commun. 2012 Aug 3;424(3):524-9. doi: 10.1016/j.bbrc.2012.06.149. Epub 2012 Jul 6. Biochem Biophys Res Commun. 2012. PMID: 22771328
-
Hacking RNA: Hakai promotes tumorigenesis by enhancing the RNA-binding function of PSF.Cell Cycle. 2009 Nov 15;8(22):3648-51. doi: 10.4161/cc.8.22.9909. Epub 2009 Nov 24. Cell Cycle. 2009. PMID: 19855157 Free PMC article. Review.
-
PPARgamma physiology and pathology in gastrointestinal epithelial cells.Mol Cells. 2007 Oct 31;24(2):167-76. Mol Cells. 2007. PMID: 17978568 Review.
Cited by
-
Development and validation of a DNA repair gene signature for prognosis prediction in Colon Cancer.J Cancer. 2020 Aug 12;11(20):5918-5928. doi: 10.7150/jca.46328. eCollection 2020. J Cancer. 2020. PMID: 32922534 Free PMC article.
-
Kub5-Hera, the human Rtt103 homolog, plays dual functional roles in transcription termination and DNA repair.Nucleic Acids Res. 2014 Apr;42(8):4996-5006. doi: 10.1093/nar/gku160. Epub 2014 Mar 3. Nucleic Acids Res. 2014. PMID: 24589584 Free PMC article.
-
Delineating the role of nuclear receptors in colorectal cancer, a focused review.Discov Oncol. 2024 Feb 19;15(1):41. doi: 10.1007/s12672-023-00808-x. Discov Oncol. 2024. PMID: 38372868 Free PMC article. Review.
-
Characterization of MNK1b DNA Aptamers That Inhibit Proliferation in MDA-MB231 Breast Cancer Cells.Mol Ther Nucleic Acids. 2016 Jan 5;5(1):e275. doi: 10.1038/mtna.2015.50. Mol Ther Nucleic Acids. 2016. PMID: 26730812 Free PMC article.
-
The MNK1/2-eIF4E Axis as a Potential Therapeutic _target in Melanoma.Int J Mol Sci. 2020 Jun 5;21(11):4055. doi: 10.3390/ijms21114055. Int J Mol Sci. 2020. PMID: 32517051 Free PMC article. Review.
References
-
- Siegel R, Naishadham D, Jemal A (2012) Cancer statistics, 2012. CA Cancer J Clin 62: 10–29. - PubMed
-
- Goldberg RM (2006) Therapy for metastatic colorectal cancer. Oncologist 11: 981–987. - PubMed
-
- Issemann I, Green S (1990) Activation of a member of the steroid hormone receptor superfamily by peroxisome proliferators. Nature 347: 645–650. - PubMed
-
- Evans RM (2005) The nuclear receptor superfamily: a rosetta stone for physiology. Mol Endocrinol 19: 1429–1438. - PubMed
Publication types
MeSH terms
Substances
Grants and funding
LinkOut - more resources
Full Text Sources
Other Literature Sources
Research Materials
Miscellaneous