Alternate virtual populations elucidate the type I interferon signature predictive of the response to rituximab in rheumatoid arthritis
- PMID: 23841912
- PMCID: PMC3717130
- DOI: 10.1186/1471-2105-14-221
Alternate virtual populations elucidate the type I interferon signature predictive of the response to rituximab in rheumatoid arthritis
Abstract
Background: Mechanistic biosimulation can be used in drug development to form testable hypotheses, develop predictions of efficacy before clinical trial results are available, and elucidate clinical response to therapy. However, there is a lack of tools to simultaneously (1) calibrate the prevalence of mechanistically distinct, large sets of virtual patients so their simulated responses statistically match phenotypic variability reported in published clinical trial outcomes, and (2) explore alternate hypotheses of those prevalence weightings to reflect underlying uncertainty in population biology. Here, we report the development of an algorithm, MAPEL (Mechanistic Axes Population Ensemble Linkage), which utilizes a mechanistically-based weighting method to match clinical trial statistics. MAPEL is the first algorithm for developing weighted virtual populations based on biosimulation results that enables the rapid development of an ensemble of alternate virtual population hypotheses, each validated by a composite goodness-of-fit criterion.
Results: Virtual patient cohort mechanistic biosimulation results were successfully calibrated with an acceptable composite goodness-of-fit to clinical populations across multiple therapeutic interventions. The resulting virtual populations were employed to investigate the mechanistic underpinnings of variations in the response to rituximab. A comparison between virtual populations with a strong or weak American College of Rheumatology (ACR) score in response to rituximab suggested that interferon β (IFNβ) was an important mechanistic contributor to the disease state, a signature that has previously been identified though the underlying mechanisms remain unclear. Sensitivity analysis elucidated key anti-inflammatory properties of IFNβ that modulated the pathophysiologic state, consistent with the observed prognostic correlation of baseline type I interferon measurements with clinical response. Specifically, the effects of IFNβ on proliferation of fibroblast-like synoviocytes and interleukin-10 synthesis in macrophages each partially counteract reductions in synovial inflammation imparted by rituximab. A multianalyte biomarker panel predictive for virtual population therapeutic responses suggested population dependencies on B cell-dependent mediators as well as additional markers implicating fibroblast-like synoviocytes.
Conclusions: The results illustrate how the MAPEL algorithm can leverage knowledge of cellular and molecular function through biosimulation to propose clear mechanistic hypotheses for differences in clinical populations. Furthermore, MAPEL facilitates the development of multianalyte biomarkers prognostic of patient responses in silico.
Figures
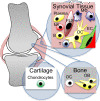
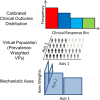
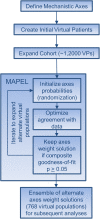
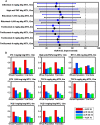
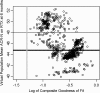
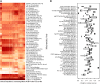
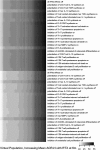
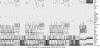
Similar articles
-
Pretreatment synovial transcriptional profile is associated with early and late clinical response in rheumatoid arthritis patients treated with rituximab.Ann Rheum Dis. 2012 Nov;71(11):1888-94. doi: 10.1136/annrheumdis-2011-201115. Epub 2012 Jun 26. Ann Rheum Dis. 2012. PMID: 22736099
-
Assessment of rituximab's immunomodulatory synovial effects (ARISE trial). 1: clinical and synovial biomarker results.Ann Rheum Dis. 2008 Mar;67(3):402-8. doi: 10.1136/ard.2007.074229. Epub 2007 Jul 20. Ann Rheum Dis. 2008. PMID: 17644541 Free PMC article. Clinical Trial.
-
B cell-_targeted therapy for rheumatoid arthritis: an update on the evidence.Drugs. 2006;66(5):625-39. doi: 10.2165/00003495-200666050-00004. Drugs. 2006. PMID: 16620141 Review.
-
Inflammation and autoantibody markers identify rheumatoid arthritis patients with enhanced clinical benefit following rituximab treatment.Arthritis Rheum. 2011 Dec;63(12):3681-91. doi: 10.1002/art.30596. Arthritis Rheum. 2011. PMID: 22127691 Clinical Trial.
-
Biologic therapy for rheumatoid arthritis: clinical efficacy and predictors of response.BioDrugs. 2009;23(2):111-24. doi: 10.2165/00063030-200923020-00004. BioDrugs. 2009. PMID: 19489652 Review.
Cited by
-
A Six-Stage Workflow for Robust Application of Systems Pharmacology.CPT Pharmacometrics Syst Pharmacol. 2016 May;5(5):235-49. doi: 10.1002/psp4.12071. Epub 2016 Apr 16. CPT Pharmacometrics Syst Pharmacol. 2016. PMID: 27299936 Free PMC article.
-
Applications of Quantitative Systems Pharmacology in Model-Informed Drug Discovery: Perspective on Impact and Opportunities.CPT Pharmacometrics Syst Pharmacol. 2019 Nov;8(11):777-791. doi: 10.1002/psp4.12463. Epub 2019 Oct 25. CPT Pharmacometrics Syst Pharmacol. 2019. PMID: 31535440 Free PMC article.
-
Linear-In-Flux-Expressions Methodology: Toward a Robust Mathematical Framework for Quantitative Systems Pharmacology Simulators.Gene Regul Syst Bio. 2017 Jul 26;11:1177625017711414. doi: 10.1177/1177625017711414. eCollection 2017. Gene Regul Syst Bio. 2017. PMID: 29581702 Free PMC article. Review.
-
The Virtual Anemia Trial: An Assessment of Model-Based In Silico Clinical Trials of Anemia Treatment Algorithms in Patients With Hemodialysis.CPT Pharmacometrics Syst Pharmacol. 2018 Apr;7(4):219-227. doi: 10.1002/psp4.12276. Epub 2018 Jan 31. CPT Pharmacometrics Syst Pharmacol. 2018. PMID: 29368434 Free PMC article.
-
Multiscale, mechanistic model of Rheumatoid Arthritis to enable decision making in late stage drug development.NPJ Syst Biol Appl. 2024 Nov 4;10(1):126. doi: 10.1038/s41540-024-00454-1. NPJ Syst Biol Appl. 2024. PMID: 39496637 Free PMC article.
References
-
- Aletaha D, Neogi T, Silman AJ, Funovits J, Felson DT, Bingham CO, Birnbaum NS, Burmester GR, Bykerk VP, Cohen MD, Combe B, Costenbader KH, Dougados M, Emery P, Ferraccioli G, Hazes JMW, Hobbs K, Huizinga TWJ, Kavanaugh A, Kay J, Kvien TK, Laing T, Mease P, Ménard HA, Moreland LW, Naden RL, Pincus T, Smolen JS, Stanislawska-Biernat E, Symmons D. et al.Rheumatoid arthritis classification criteria: an American College of Rheumatology/European League Against Rheumatism collaborative initiative. Arthritis & rheum. 2010;62:2569–81. doi: 10.1002/art.27584. - DOI - PubMed
Publication types
MeSH terms
Substances
LinkOut - more resources
Full Text Sources
Other Literature Sources
Medical