Cancer gene discovery: exploiting insertional mutagenesis
- PMID: 23928056
- PMCID: PMC3836224
- DOI: 10.1158/1541-7786.MCR-13-0244
Cancer gene discovery: exploiting insertional mutagenesis
Abstract
Insertional mutagenesis has been used as a functional forward genetics screen for the identification of novel genes involved in the pathogenesis of human cancers. Different insertional mutagens have been successfully used to reveal new cancer genes. For example, retroviruses are integrating viruses with the capacity to induce the deregulation of genes in the neighborhood of the insertion site. Retroviruses have been used for more than 30 years to identify cancer genes in the hematopoietic system and mammary gland. Similarly, another tool that has revolutionized cancer gene discovery is the cut-and-paste transposons. These DNA elements have been engineered to contain strong promoters and stop cassettes that may function to perturb gene expression upon integration proximal to genes. In addition, complex mouse models characterized by tissue-restricted activity of transposons have been developed to identify oncogenes and tumor suppressor genes that control the development of a wide range of solid tumor types, extending beyond those tissues accessible using retrovirus-based approaches. Most recently, lentiviral vectors have appeared on the scene for use in cancer gene screens. Lentiviral vectors are replication-defective integrating vectors that have the advantage of being able to infect nondividing cells, in a wide range of cell types and tissues. In this review, we describe the various insertional mutagens focusing on their advantages/limitations, and we discuss the new and promising tools that will improve the insertional mutagenesis screens of the future.
Figures
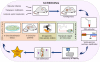
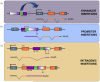
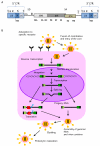
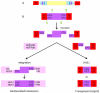
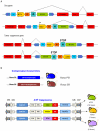
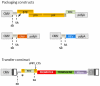
Similar articles
-
Retroviral insertional mutagenesis: past, present and future.Oncogene. 2005 Nov 21;24(52):7656-72. doi: 10.1038/sj.onc.1209043. Oncogene. 2005. PMID: 16299527 Review.
-
Cancer gene discovery in solid tumours using transposon-based somatic mutagenesis in the mouse.Nature. 2005 Jul 14;436(7048):272-6. doi: 10.1038/nature03681. Nature. 2005. PMID: 16015333
-
The use of DNA transposons for cancer gene discovery in mice.Methods Enzymol. 2010;477:91-106. doi: 10.1016/S0076-6879(10)77006-3. Methods Enzymol. 2010. PMID: 20699138
-
The utility of transposon mutagenesis for cancer studies in the era of genome editing.Genome Biol. 2015 Oct 19;16:229. doi: 10.1186/s13059-015-0794-y. Genome Biol. 2015. PMID: 26481584 Free PMC article. Review.
-
A novel approach to identify driver genes involved in androgen-independent prostate cancer.Mol Cancer. 2014 May 23;13:120. doi: 10.1186/1476-4598-13-120. Mol Cancer. 2014. PMID: 24885513 Free PMC article.
Cited by
-
Arid1a Loss Enhances Disease Progression in a Murine Model of Osteosarcoma.Cancers (Basel). 2024 Jul 31;16(15):2725. doi: 10.3390/cancers16152725. Cancers (Basel). 2024. PMID: 39123453 Free PMC article.
-
WDR26 and MTF2 are therapeutic _targets in multiple myeloma.J Hematol Oncol. 2021 Dec 7;14(1):203. doi: 10.1186/s13045-021-01217-9. J Hematol Oncol. 2021. PMID: 34876184 Free PMC article.
-
Induced Pluripotent Stem Cells (iPSCs) and Gene Therapy: A New Era for the Treatment of Neurological Diseases.Int J Mol Sci. 2021 Dec 20;22(24):13674. doi: 10.3390/ijms222413674. Int J Mol Sci. 2021. PMID: 34948465 Free PMC article. Review.
-
VISPA: a computational pipeline for the identification and analysis of genomic vector integration sites.Genome Med. 2014 Sep 3;6(9):67. doi: 10.1186/s13073-014-0067-5. eCollection 2014. Genome Med. 2014. PMID: 25342980 Free PMC article.
-
GENE-IS: Time-Efficient and Accurate Analysis of Viral Integration Events in Large-Scale Gene Therapy Data.Mol Ther Nucleic Acids. 2017 Mar 17;6:133-139. doi: 10.1016/j.omtn.2016.12.001. Epub 2016 Dec 10. Mol Ther Nucleic Acids. 2017. PMID: 28325279 Free PMC article.
References
-
- Uren AG, Kool J, Berns A, van Lohuizen M. Retroviral insertional mutagenesis: past, present and future. Oncogene. 2005;24:7656–72. - PubMed
-
- Morishita K, Parker DS, Mucenski ML, Jenkins NA, Copeland NG, Ihle JN. Retroviral activation of a novel gene encoding a zinc finger protein in IL-3-dependent myeloid leukemia cell lines. Cell. 1988;54:831–40. - PubMed
-
- Kool J, Berns A. High-throughput insertional mutagenesis screens in mice to identify oncogenic networks. Nature reviews Cancer. 2009;9:389–99. - PubMed
Publication types
MeSH terms
Substances
Grants and funding
LinkOut - more resources
Full Text Sources
Other Literature Sources
Molecular Biology Databases