Metal preferences and metallation
- PMID: 25160626
- PMCID: PMC4192464
- DOI: 10.1074/jbc.R114.588145
Metal preferences and metallation
Abstract
The metal binding preferences of most metalloproteins do not match their metal requirements. Thus, metallation of an estimated 30% of metalloenzymes is aided by metal delivery systems, with ∼ 25% acquiring preassembled metal cofactors. The remaining ∼ 70% are presumed to compete for metals from buffered metal pools. Metallation is further aided by maintaining the relative concentrations of these pools as an inverse function of the stabilities of the respective metal complexes. For example, magnesium enzymes always prefer to bind zinc, and these metals dominate the metalloenzymes without metal delivery systems. Therefore, the buffered concentration of zinc is held at least a million-fold below magnesium inside most cells.
Keywords: Copper; Iron; Irving-Williams Series; Manganese; Metal Sensors; Metallochaperone; Metalloenzymes; Nickel; Polydisperse Buffer; Zinc.
© 2014 by The American Society for Biochemistry and Molecular Biology, Inc.
Figures
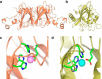
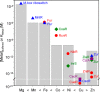
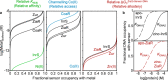

Similar articles
-
The mismetallation of enzymes during oxidative stress.J Biol Chem. 2014 Oct 10;289(41):28121-8. doi: 10.1074/jbc.R114.588814. Epub 2014 Aug 26. J Biol Chem. 2014. PMID: 25160623 Free PMC article. Review.
-
Thematic minireview series: metals in biology 2014.J Biol Chem. 2014 Oct 10;289(41):28094. doi: 10.1074/jbc.R114.607093. Epub 2014 Aug 26. J Biol Chem. 2014. PMID: 25160633 Free PMC article.
-
Metallation and mismetallation of iron and manganese proteins in vitro and in vivo: the class I ribonucleotide reductases as a case study.Metallomics. 2012 Oct;4(10):1020-36. doi: 10.1039/c2mt20142a. Epub 2012 Sep 18. Metallomics. 2012. PMID: 22991063 Free PMC article. Review.
-
Direct observation of structurally encoded metal discrimination and ether bond formation in a heterodinuclear metalloprotein.Proc Natl Acad Sci U S A. 2013 Oct 22;110(43):17189-94. doi: 10.1073/pnas.1304368110. Epub 2013 Oct 7. Proc Natl Acad Sci U S A. 2013. PMID: 24101498 Free PMC article.
-
Specificity of metal sensing: iron and manganese homeostasis in Bacillus subtilis.J Biol Chem. 2014 Oct 10;289(41):28112-20. doi: 10.1074/jbc.R114.587071. Epub 2014 Aug 26. J Biol Chem. 2014. PMID: 25160631 Free PMC article. Review.
Cited by
-
Zinc homeostasis in Pseudomonas.Biometals. 2023 Aug;36(4):729-744. doi: 10.1007/s10534-022-00475-5. Epub 2022 Dec 6. Biometals. 2023. PMID: 36472780 Free PMC article. Review.
-
Allosteric control of metal-responsive transcriptional regulators in bacteria.J Biol Chem. 2020 Feb 7;295(6):1673-1684. doi: 10.1074/jbc.REV119.011444. Epub 2019 Dec 19. J Biol Chem. 2020. PMID: 31857375 Free PMC article. Review.
-
Proteome-wide Analysis of Protein Thermal Stability in the Model Higher Plant Arabidopsis thaliana.Mol Cell Proteomics. 2019 Feb;18(2):308-319. doi: 10.1074/mcp.RA118.001124. Epub 2018 Nov 6. Mol Cell Proteomics. 2019. PMID: 30401684 Free PMC article.
-
Extracellular Electron Transfer Powers Enterococcus faecalis Biofilm Metabolism.mBio. 2018 Apr 10;9(2):e00626-17. doi: 10.1128/mBio.00626-17. mBio. 2018. PMID: 29636430 Free PMC article.
-
Survival of Anaerobic Fe2+ Stress Requires the ClpXP Protease.J Bacteriol. 2018 Mar 26;200(8):e00671-17. doi: 10.1128/JB.00671-17. Print 2018 Apr 15. J Bacteriol. 2018. PMID: 29378887 Free PMC article.
References
-
- Irving H., Williams R. J. (1948) Order of stability of metal complexes. Nature 162, 746–747
-
- Johnson D. A., Nelson P. G. (1995) Factors determining the ligand field stabilization energies of the hexaaqua 2+ complexes of the first transition series and the Irving-Williams order. Inorg. Chem. 34, 5666–5671 - PubMed
-
- Schalk I. J., Yue W. W., Buchanan S. K. (2004) Recognition of iron-free siderophores by TonB-dependent iron transporters. Mol. Microbiol. 54, 14–22 - PubMed
-
- Parker Siburt C. J., Mietzner T. A., Crumbliss A. L. (2012) FbpA — a bacterial transferrin with more to offer. Biochim. Biophys. Acta 1820, 379–392 - PubMed
Publication types
MeSH terms
Substances
Associated data
- Actions
- Actions
Grants and funding
LinkOut - more resources
Full Text Sources
Other Literature Sources
Medical