Nitric oxide signaling is recruited as a compensatory mechanism for sustaining synaptic plasticity in Alzheimer's disease mice
- PMID: 25926464
- PMCID: PMC4412902
- DOI: 10.1523/JNEUROSCI.4002-14.2015
Nitric oxide signaling is recruited as a compensatory mechanism for sustaining synaptic plasticity in Alzheimer's disease mice
Abstract
Synaptic plasticity deficits are increasingly recognized as causing the memory impairments which define Alzheimer's disease (AD). In AD mouse models, evidence of abnormal synaptic function is present before the onset of cognitive deficits, and presents as increased synaptic depression revealed only when synaptic homeostasis is challenged, such as with suppression of ryanodine receptor (RyR)-evoked calcium signaling. Otherwise, at early disease stages, the synaptic physiology phenotype appears normal. This suggests compensatory mechanisms are recruited to maintain a functionally normal net output of the hippocampal circuit. A candidate calcium-regulated synaptic modulator is nitric oxide (NO), which acts presynaptically to boost vesicle release and glutamatergic transmission. Here we tested whether there is a feedforward cycle between the increased RyR calcium release seen in presymptomatic AD mice and aberrant NO signaling which augments synaptic plasticity. Using a combination of electrophysiological approaches, two-photon calcium imaging, and protein biochemistry in hippocampal tissue from presymptomatic 3xTg-AD and NonTg mice, we show that blocking NO synthesis results in markedly augmented synaptic depression mediated through presynaptic mechanisms in 3xTg-AD mice. Additionally, blocking NO reduces the augmented synaptically evoked dendritic calcium release mediated by enhanced RyR calcium release. This is accompanied by increased nNOS levels in the AD mice and is reversed upon normalization of RyR-evoked calcium release with chronic dantrolene treatment. Thus, recruitment of NO is serving a compensatory role to boost synaptic transmission and plasticity during early AD stages. However, NO's dual role in neuroprotection and neurodegeneration may convert to maladaptive functions as the disease progresses.
Keywords: calcium; homeostasis; nitric oxide; ryanodine receptor; synaptic depression; synaptic plasticity.
Copyright © 2015 the authors 0270-6474/15/356893-10$15.00/0.
Figures
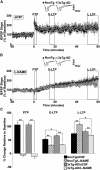
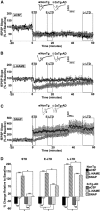
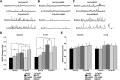
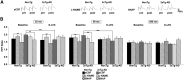
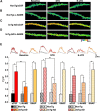
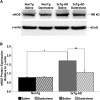
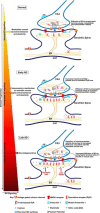
Similar articles
-
NMDA-mediated Ca(2+) influx drives aberrant ryanodine receptor activation in dendrites of young Alzheimer's disease mice.J Neurosci. 2010 Sep 8;30(36):12128-37. doi: 10.1523/JNEUROSCI.2474-10.2010. J Neurosci. 2010. PMID: 20826675 Free PMC article.
-
Deviant ryanodine receptor-mediated calcium release resets synaptic homeostasis in presymptomatic 3xTg-AD mice.J Neurosci. 2009 Jul 29;29(30):9458-70. doi: 10.1523/JNEUROSCI.2047-09.2009. J Neurosci. 2009. PMID: 19641109 Free PMC article.
-
Early presynaptic and postsynaptic calcium signaling abnormalities mask underlying synaptic depression in presymptomatic Alzheimer's disease mice.J Neurosci. 2012 Jun 13;32(24):8341-53. doi: 10.1523/JNEUROSCI.0936-12.2012. J Neurosci. 2012. PMID: 22699914 Free PMC article.
-
Hippocampal synaptic plasticity in Alzheimer's disease: what have we learned so far from transgenic models?Rev Neurosci. 2011;22(4):373-402. doi: 10.1515/RNS.2011.035. Epub 2011 Jul 6. Rev Neurosci. 2011. PMID: 21732714 Review.
-
Contribution of Ca2+ release channels to hippocampal synaptic plasticity and spatial memory: potential redox modulation.Antioxid Redox Signal. 2014 Aug 20;21(6):892-914. doi: 10.1089/ars.2013.5796. Epub 2014 Mar 11. Antioxid Redox Signal. 2014. PMID: 24410659 Review.
Cited by
-
Potential mechanisms underlying lithium treatment for Alzheimer's disease and COVID-19.Eur Rev Med Pharmacol Sci. 2022 Mar;26(6):2201-2214. doi: 10.26355/eurrev_202203_28369. Eur Rev Med Pharmacol Sci. 2022. PMID: 35363371 Free PMC article. Review.
-
Nitric Oxide: Exploring the Contextual Link with Alzheimer's Disease.Oxid Med Cell Longev. 2016;2016:7205747. doi: 10.1155/2016/7205747. Epub 2016 Dec 21. Oxid Med Cell Longev. 2016. PMID: 28096943 Free PMC article. Review.
-
Neuroprotective effects of apigenin against inflammation, neuronal excitability and apoptosis in an induced pluripotent stem cell model of Alzheimer's disease.Sci Rep. 2016 Aug 12;6:31450. doi: 10.1038/srep31450. Sci Rep. 2016. PMID: 27514990 Free PMC article.
-
Synaptic and memory dysfunction induced by tau oligomers is rescued by up-regulation of the nitric oxide cascade.Mol Neurodegener. 2019 Jun 27;14(1):26. doi: 10.1186/s13024-019-0326-4. Mol Neurodegener. 2019. PMID: 31248451 Free PMC article.
-
Roles of Gasotransmitters in Synaptic Plasticity and Neuropsychiatric Conditions.Neural Plast. 2018 May 6;2018:1824713. doi: 10.1155/2018/1824713. eCollection 2018. Neural Plast. 2018. PMID: 29853837 Free PMC article. Review.
References
Publication types
MeSH terms
Substances
Grants and funding
LinkOut - more resources
Full Text Sources
Other Literature Sources
Medical