The Warburg effect: a balance of flux analysis
- PMID: 26207106
- PMCID: PMC4507278
- DOI: 10.1007/s11306-014-0760-9
The Warburg effect: a balance of flux analysis
Abstract
Cancer metabolism is characterized by increased macromolecular syntheses through coordinated increases in energy and substrate metabolism. The observation that cancer cells produce lactate in an environment of oxygen sufficiency (aerobic glycolysis) is a central theme of cancer metabolism known as the Warburg effect. Aerobic glycolysis in cancer metabolism is accompanied by increased pentose cycle and anaplerotic activities producing energy and substrates for macromolecular synthesis. How these processes are coordinated is poorly understood. Recent advances have focused on molecular regulation of cancer metabolism by oncogenes and tumor suppressor genes which regulate numerous enzymatic steps of central glucose metabolism. In the past decade, new insights in cancer metabolism have emerged through the application of stable isotopes particularly from 13C carbon tracing. Such studies have provided new evidence for system-wide changes in cancer metabolism in response to chemotherapy. Interestingly, experiments using metabolic inhibitors on individual biochemical pathways all demonstrate similar system-wide effects on cancer metabolism as in _targeted therapies. Since biochemical reactions in the Warburg effect place competing demands on available precursors, high energy phosphates and reducing equivalents, the cancer metabolic system must fulfill the condition of balance of flux (homeostasis). In this review, the functions of the pentose cycle and of the tricarboxylic acid (TCA) cycle in cancer metabolism are analyzed from the balance of flux point of view. Anticancer treatments that _target molecular signaling pathways or inhibit metabolism alter the invasive or proliferative behavior of the cancer cells by their effects on the balance of flux (homeostasis) of the cancer metabolic phenotype.
Conflict of interest statement
Disclosure statement: The authors have no conflicts of interest to disclose.
Figures
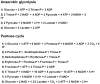
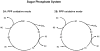
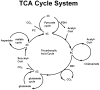
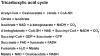
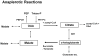
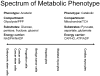
Similar articles
-
Updates to a 13C metabolic flux analysis model for evaluating energy metabolism in cultured cerebellar granule neurons from neonatal rats.Neurochem Int. 2017 Oct;109:54-67. doi: 10.1016/j.neuint.2017.03.020. Epub 2017 Apr 13. Neurochem Int. 2017. PMID: 28412312 Free PMC article.
-
The Warburg effect: essential part of metabolic reprogramming and central contributor to cancer progression.Int J Radiat Biol. 2019 Jul;95(7):912-919. doi: 10.1080/09553002.2019.1589653. Epub 2019 Mar 22. Int J Radiat Biol. 2019. PMID: 30822194 Review.
-
Mechanisms of Metabolic Reprogramming in Cancer Cells Supporting Enhanced Growth and Proliferation.Cells. 2021 Apr 29;10(5):1056. doi: 10.3390/cells10051056. Cells. 2021. PMID: 33946927 Free PMC article. Review.
-
Regulation of Aerobic Glycolysis by microRNAs in Cancer.Mol Cell Pharmacol. 2011;3(3):125-134. Mol Cell Pharmacol. 2011. PMID: 22792411 Free PMC article.
-
[Isotopic tracing technique in quantitative analysis of metabolic reprogramming in tumor cells].Se Pu. 2015 Feb;33(2):112-5. doi: 10.3724/sp.j.1123.2014.11011. Se Pu. 2015. PMID: 25989681 Review. Chinese.
Cited by
-
Research progress on the mechanism of glycolysis in ovarian cancer.Front Immunol. 2023 Nov 28;14:1284853. doi: 10.3389/fimmu.2023.1284853. eCollection 2023. Front Immunol. 2023. PMID: 38090580 Free PMC article. Review.
-
circ-CFH promotes the development of HCC by regulating cell proliferation, apoptosis, migration, invasion, and glycolysis through the miR-377-3p/RNF38 axis.Open Life Sci. 2022 Mar 23;17(1):248-260. doi: 10.1515/biol-2022-0029. eCollection 2022. Open Life Sci. 2022. PMID: 35415236 Free PMC article.
-
Defining Metabolic Rewiring in Lung Squamous Cell Carcinoma.Metabolites. 2019 Mar 7;9(3):47. doi: 10.3390/metabo9030047. Metabolites. 2019. PMID: 30866469 Free PMC article.
-
Exploring the impact of circRNAs on cancer glycolysis: Insights into tumor progression and therapeutic strategies.Noncoding RNA Res. 2024 May 5;9(3):970-994. doi: 10.1016/j.ncrna.2024.05.001. eCollection 2024 Sep. Noncoding RNA Res. 2024. PMID: 38770106 Free PMC article. Review.
-
A Time-Course Comparison of Skeletal Muscle Metabolomic Alterations in Walker-256 Tumour-Bearing Rats at Different Stages of Life.Metabolites. 2021 Jun 20;11(6):404. doi: 10.3390/metabo11060404. Metabolites. 2021. PMID: 34202988 Free PMC article.
References
-
- Boren J, et al. Gleevec (STI571) Influences Metabolic Enzyme Activities and Glucose Carbon Flow toward Nucleic Acid and Fatty Acid Synthesis in Myeloid Tumor Cells. Journal of Biological Chemistry. 2001;276:37747–37753. - PubMed
-
- Boros LG, Bassilian S, Lim S, Lee WN. Genistein inhibits nonoxidative ribose synthesis in MIA pancreatic adenocarcinoma cells: a new mechanism of controlling tumor growth. Pancreas. 2001;22:1–7. - PubMed
-
- Boros LG, Cascante M, Lee WN. Metabolic profiling of cell growth and death in cancer: applications in drug discovery. Drug Discov Today. 2002;7:364–72. - PubMed
-
- Boros LG, Lee WN, Go VL. A metabolic hypothesis of cell growth and death in pancreatic cancer. Pancreas. 2002;24:26–33. - PubMed
Grants and funding
LinkOut - more resources
Full Text Sources
Other Literature Sources
Miscellaneous