Planar carbon nanotube-graphene hybrid films for high-performance broadband photodetectors
- PMID: 26446884
- PMCID: PMC4633958
- DOI: 10.1038/ncomms9589
Planar carbon nanotube-graphene hybrid films for high-performance broadband photodetectors
Abstract
Graphene has emerged as a promising material for photonic applications fuelled by its superior electronic and optical properties. However, the photoresponsivity is limited by the low absorption cross-section and ultrafast recombination rates of photoexcited carriers. Here we demonstrate a photoconductive gain of ∼10(5) electrons per photon in a carbon nanotube-graphene hybrid due to efficient photocarriers generation and transport within the nanostructure. A broadband photodetector (covering 400-1,550 nm) based on such hybrid films is fabricated with a high photoresponsivity of >100 A W(-1) and a fast response time of ∼100 μs. The combination of ultra-broad bandwidth, high responsivities and fast operating speeds affords new opportunities for facile and scalable fabrication of all-carbon optoelectronic devices.
Conflict of interest statement
F.W., Y.D.L., Y.L, Y.X. and R.Z have filed a Chinese patent based on this work (application no. 201410265599.1).
Figures
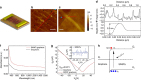
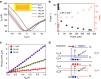
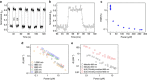
Similar articles
-
Broadband high photoresponse from pure monolayer graphene photodetector.Nat Commun. 2013;4:1811. doi: 10.1038/ncomms2830. Nat Commun. 2013. PMID: 23651999
-
Light Trapping in Conformal Graphene/Silicon Nanoholes for High-Performance Photodetectors.ACS Appl Mater Interfaces. 2019 Aug 21;11(33):30421-30429. doi: 10.1021/acsami.9b08268. Epub 2019 Aug 6. ACS Appl Mater Interfaces. 2019. PMID: 31342736
-
Sensitive and Robust Ultraviolet Photodetector Array Based on Self-Assembled Graphene/C60 Hybrid Films.ACS Appl Mater Interfaces. 2018 Nov 7;10(44):38326-38333. doi: 10.1021/acsami.8b11596. Epub 2018 Sep 20. ACS Appl Mater Interfaces. 2018. PMID: 30207446
-
Graphene Hybrid Structures for Integrated and Flexible Optoelectronics.Adv Mater. 2020 Jul;32(27):e1902039. doi: 10.1002/adma.201902039. Epub 2019 Jul 8. Adv Mater. 2020. PMID: 31282020 Review.
-
Graphene-Based Light Sensing: Fabrication, Characterisation, Physical Properties and Performance.Materials (Basel). 2018 Sep 18;11(9):1762. doi: 10.3390/ma11091762. Materials (Basel). 2018. PMID: 30231517 Free PMC article. Review.
Cited by
-
New 2D graphene hybrid composites as an effective base element of optical nanodevices.Beilstein J Nanotechnol. 2018 Apr 30;9:1321-1327. doi: 10.3762/bjnano.9.125. eCollection 2018. Beilstein J Nanotechnol. 2018. PMID: 29977667 Free PMC article.
-
Graphene-Based Semiconductor Heterostructures for Photodetectors.Micromachines (Basel). 2018 Jul 13;9(7):350. doi: 10.3390/mi9070350. Micromachines (Basel). 2018. PMID: 30424283 Free PMC article. Review.
-
Thin Graphene-Nanotube Films for Electronic and Photovoltaic Devices: DFTB Modeling.Membranes (Basel). 2020 Nov 13;10(11):341. doi: 10.3390/membranes10110341. Membranes (Basel). 2020. PMID: 33202838 Free PMC article.
-
Van Hove Singularity-Enhanced Raman Scattering and Photocurrent Generation in Twisted Monolayer-Bilayer Graphene.ACS Nano. 2024 Sep 10;18(36):25183-25192. doi: 10.1021/acsnano.4c07302. Epub 2024 Aug 29. ACS Nano. 2024. PMID: 39207384
-
Broadband Tunable, Polarization-Selective and Directional Emission of (6,5) Carbon Nanotubes Coupled to Plasmonic Crystals.Nano Lett. 2016 May 11;16(5):3278-84. doi: 10.1021/acs.nanolett.6b00827. Epub 2016 Apr 25. Nano Lett. 2016. PMID: 27105249 Free PMC article.
References
-
- Geim A. & Grigorieva I. Van der Waals heterostructures. Nature 499, 419–425 (2013). - PubMed
-
- Lv R., Cruz-Silva E. & Terrones M. Building complex hybrid carbon architectures by covalent interconnections: graphene-nanotube hybrids and more. ACS Nano 8, 4061–4069 (2014). - PubMed
-
- Dimitrakakis G. K., Tylianakis E. & Froudakis G. E. Pillared graphene: a new 3-D network nanostructure for enhanced hydrogen storage. Nano Lett. 8, 3166–3170 (2008). - PubMed
-
- Paul R. K. et al.. Synthesis of a Pillared Graphene Nanostructure: A Counterpart of Three-Dimensional Carbon Architectures. Small. 6, 2309–2313 (2010). - PubMed
-
- Zhu Y. et al.. A seamless three-dimensional carbon nanotube graphene hybrid material. Nat. Commun. 3, 1225 (2012). - PubMed
Publication types
LinkOut - more resources
Full Text Sources
Other Literature Sources