Metabolic Symbiosis Enables Adaptive Resistance to Anti-angiogenic Therapy that Is Dependent on mTOR Signaling
- PMID: 27134166
- PMCID: PMC4872464
- DOI: 10.1016/j.celrep.2016.04.029
Metabolic Symbiosis Enables Adaptive Resistance to Anti-angiogenic Therapy that Is Dependent on mTOR Signaling
Abstract
Therapeutic _targeting of tumor angiogenesis with VEGF inhibitors results in demonstrable, but transitory efficacy in certain human tumors and mouse models of cancer, limited by unconventional forms of adaptive/evasive resistance. In one such mouse model, potent angiogenesis inhibitors elicit compartmental reorganization of cancer cells around remaining blood vessels. The glucose and lactate transporters GLUT1 and MCT4 are induced in distal hypoxic cells in a HIF1α-dependent fashion, indicative of glycolysis. Tumor cells proximal to blood vessels instead express the lactate transporter MCT1, and p-S6, the latter reflecting mTOR signaling. Normoxic cancer cells import and metabolize lactate, resulting in upregulation of mTOR signaling via glutamine metabolism enhanced by lactate catabolism. Thus, metabolic symbiosis is established in the face of angiogenesis inhibition, whereby hypoxic cancer cells import glucose and export lactate, while normoxic cells import and catabolize lactate. mTOR signaling inhibition disrupts this metabolic symbiosis, associated with upregulation of the glucose transporter GLUT2.
Copyright © 2016 The Authors. Published by Elsevier Inc. All rights reserved.
Figures
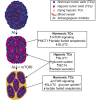
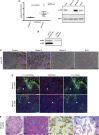
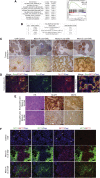
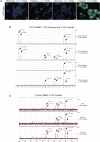
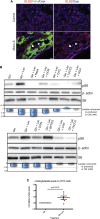
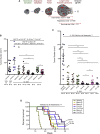
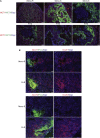
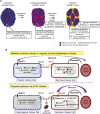
Similar articles
-
Antitumor effect of everolimus in preclinical models of high-grade gastroenteropancreatic neuroendocrine carcinomas.Neuroendocrinology. 2013;97(4):331-40. doi: 10.1159/000347063. Epub 2013 May 22. Neuroendocrinology. 2013. PMID: 23343749
-
_targeting Focal Adhesion Kinase and Resistance to mTOR Inhibition in Pancreatic Neuroendocrine Tumors.J Natl Cancer Inst. 2015 May 12;107(8):djv123. doi: 10.1093/jnci/djv123. Print 2015 Aug. J Natl Cancer Inst. 2015. PMID: 25971297 Free PMC article.
-
_targeting Metabolic Symbiosis to Overcome Resistance to Anti-angiogenic Therapy.Cell Rep. 2016 May 10;15(6):1161-74. doi: 10.1016/j.celrep.2016.04.028. Epub 2016 Apr 28. Cell Rep. 2016. PMID: 27134168 Free PMC article.
-
mTOR Pathway in Gastroenteropancreatic Neuroendocrine Tumor (GEP-NETs).Front Endocrinol (Lausanne). 2020 Nov 16;11:562505. doi: 10.3389/fendo.2020.562505. eCollection 2020. Front Endocrinol (Lausanne). 2020. PMID: 33304317 Free PMC article. Review.
-
Resistance to _targeted treatment of gastroenteropancreatic neuroendocrine tumors.Endocr Relat Cancer. 2019 Mar 1;26(3):R109-R130. doi: 10.1530/ERC-18-0420. Endocr Relat Cancer. 2019. PMID: 32022503 Review.
Cited by
-
Ion Channels in Cancer: Orchestrators of Electrical Signaling and Cellular Crosstalk.Rev Physiol Biochem Pharmacol. 2022;183:103-133. doi: 10.1007/112_2020_48. Rev Physiol Biochem Pharmacol. 2022. PMID: 32894333 Review.
-
Current status and future of anti-angiogenic drugs in lung cancer.Clin Exp Med. 2023 Oct;23(6):2009-2023. doi: 10.1007/s10238-023-01039-8. Epub 2023 Mar 15. Clin Exp Med. 2023. PMID: 36920592 Review.
-
Microenvironmental regulation of tumour angiogenesis.Nat Rev Cancer. 2017 Aug;17(8):457-474. doi: 10.1038/nrc.2017.51. Epub 2017 Jul 14. Nat Rev Cancer. 2017. PMID: 28706266 Review.
-
Monocarboxylate transporters in cancer.Mol Metab. 2020 Mar;33:48-66. doi: 10.1016/j.molmet.2019.07.006. Epub 2019 Jul 27. Mol Metab. 2020. PMID: 31395464 Free PMC article. Review.
-
Killing two birds with one stone: Blocking the mitochondrial pyruvate carrier to inhibit lactate uptake by cancer cells and radiosensitize tumors.Mol Cell Oncol. 2018 May 22;5(4):e1465016. doi: 10.1080/23723556.2018.1465016. eCollection 2018. Mol Cell Oncol. 2018. PMID: 30250917 Free PMC article.
References
-
- Bergers G., Javaherian K., Lo K.M., Folkman J., Hanahan D. Effects of angiogenesis inhibitors on multistage carcinogenesis in mice. Science. 1999;284:808–812. - PubMed
-
- Casanovas O., Hicklin D.J., Bergers G., Hanahan D. Drug resistance by evasion of antiangiogenic _targeting of VEGF signaling in late-stage pancreatic islet tumors. Cancer Cell. 2005;8:299–309. - PubMed
Publication types
MeSH terms
Substances
Supplementary concepts
Grants and funding
LinkOut - more resources
Full Text Sources
Other Literature Sources
Medical
Molecular Biology Databases
Miscellaneous