Metabolic regulation of gene expression through histone acylations
- PMID: 27924077
- PMCID: PMC5320945
- DOI: 10.1038/nrm.2016.140
Metabolic regulation of gene expression through histone acylations
Abstract
Eight types of short-chain Lys acylations have recently been identified on histones: propionylation, butyrylation, 2-hydroxyisobutyrylation, succinylation, malonylation, glutarylation, crotonylation and β-hydroxybutyrylation. Emerging evidence suggests that these histone modifications affect gene expression and are structurally and functionally different from the widely studied histone Lys acetylation. In this Review, we discuss the regulation of non-acetyl histone acylation by enzymatic and metabolic mechanisms, the acylation 'reader' proteins that mediate the effects of different acylations and their physiological functions, which include signal-dependent gene activation, spermatogenesis, tissue injury and metabolic stress. We propose a model to explain our present understanding of how differential histone acylation is regulated by the metabolism of the different acyl-CoA forms, which in turn modulates the regulation of gene expression.
Figures
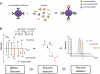
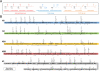
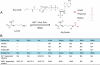
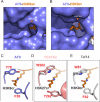
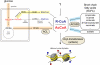
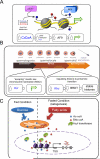
Comment in
-
HATs off for the Lasker awardees.Nat Rev Mol Cell Biol. 2018 Nov;19(11):677. doi: 10.1038/s41580-018-0065-3. Nat Rev Mol Cell Biol. 2018. PMID: 30232395 No abstract available.
Similar articles
-
Beyond histone acetylation-writing and erasing histone acylations.Curr Opin Struct Biol. 2018 Dec;53:169-177. doi: 10.1016/j.sbi.2018.10.001. Epub 2018 Nov 2. Curr Opin Struct Biol. 2018. PMID: 30391813 Review.
-
Oncometabolites drive tumorigenesis by enhancing protein acylation: from chromosomal remodelling to nonhistone modification.J Exp Clin Cancer Res. 2022 Apr 15;41(1):144. doi: 10.1186/s13046-022-02338-w. J Exp Clin Cancer Res. 2022. PMID: 35428309 Free PMC article. Review.
-
An Expanding Repertoire of Protein Acylations.Mol Cell Proteomics. 2022 Mar;21(3):100193. doi: 10.1016/j.mcpro.2022.100193. Epub 2022 Jan 6. Mol Cell Proteomics. 2022. PMID: 34999219 Free PMC article. Review.
-
Metabolically Derived Lysine Acylations and Neighboring Modifications Tune the Binding of the BET Bromodomains to Histone H4.Biochemistry. 2017 Oct 17;56(41):5485-5495. doi: 10.1021/acs.biochem.7b00595. Epub 2017 Oct 5. Biochemistry. 2017. PMID: 28945351 Free PMC article.
-
The growing landscape of lysine acetylation links metabolism and cell signalling.Nat Rev Mol Cell Biol. 2014 Aug;15(8):536-50. doi: 10.1038/nrm3841. Nat Rev Mol Cell Biol. 2014. PMID: 25053359 Review.
Cited by
-
Lactylation in cancer: Mechanisms in tumour biology and therapeutic potentials.Clin Transl Med. 2024 Nov;14(11):e70070. doi: 10.1002/ctm2.70070. Clin Transl Med. 2024. PMID: 39456119 Free PMC article. Review.
-
Lysine acetylation of cytoskeletal proteins: Emergence of an actin code.J Cell Biol. 2020 Dec 7;219(12):e202006151. doi: 10.1083/jcb.202006151. J Cell Biol. 2020. PMID: 33044556 Free PMC article. Review.
-
YiaC and CobB regulate lysine lactylation in Escherichia coli.Nat Commun. 2022 Nov 4;13(1):6628. doi: 10.1038/s41467-022-34399-y. Nat Commun. 2022. PMID: 36333310 Free PMC article.
-
Updated Mechanisms of GCN5-The Monkey King of the Plant Kingdom in Plant Development and Resistance to Abiotic Stresses.Cells. 2021 Apr 22;10(5):979. doi: 10.3390/cells10050979. Cells. 2021. PMID: 33922251 Free PMC article. Review.
-
Proteome-Wide Analyses Reveal the Diverse Functions of Lysine 2-Hydroxyisobutyrylation in Oryza sativa.Rice (N Y). 2020 Jun 5;13(1):34. doi: 10.1186/s12284-020-00389-1. Rice (N Y). 2020. PMID: 32572646 Free PMC article.
References
-
- Dawson MA, Kouzarides T. Cancer epigenetics: from mechanism to therapy. Cell. 2012;150:12–27. - PubMed
Publication types
MeSH terms
Substances
Grants and funding
LinkOut - more resources
Full Text Sources
Other Literature Sources