EXTL3 mutations cause skeletal dysplasia, immune deficiency, and developmental delay
- PMID: 28148688
- PMCID: PMC5339678
- DOI: 10.1084/jem.20161525
EXTL3 mutations cause skeletal dysplasia, immune deficiency, and developmental delay
Abstract
We studied three patients with severe skeletal dysplasia, T cell immunodeficiency, and developmental delay. Whole-exome sequencing revealed homozygous missense mutations affecting exostosin-like 3 (EXTL3), a glycosyltransferase involved in heparan sulfate (HS) biosynthesis. Patient-derived fibroblasts showed abnormal HS composition and altered fibroblast growth factor 2 signaling, which was rescued by overexpression of wild-type EXTL3 cDNA. Interleukin-2-mediated STAT5 phosphorylation in patients' lymphocytes was markedly reduced. Interbreeding of the extl3-mutant zebrafish (box) with Tg(rag2:green fluorescent protein) transgenic zebrafish revealed defective thymopoiesis, which was rescued by injection of wild-type human EXTL3 RNA. _targeted differentiation of patient-derived induced pluripotent stem cells showed a reduced expansion of lymphohematopoietic progenitor cells and defects of thymic epithelial progenitor cell differentiation. These data identify EXTL3 mutations as a novel cause of severe immune deficiency with skeletal dysplasia and developmental delay and underline a crucial role of HS in thymopoiesis and skeletal and brain development.
© 2017 Volpi et al.
Figures
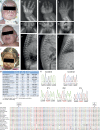
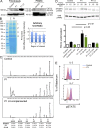
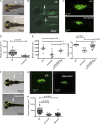
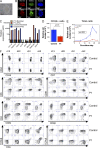
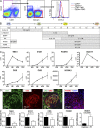
Similar articles
-
Mutations in EXTL3 Cause Neuro-immuno-skeletal Dysplasia Syndrome.Am J Hum Genet. 2017 Feb 2;100(2):281-296. doi: 10.1016/j.ajhg.2017.01.013. Epub 2017 Jan 26. Am J Hum Genet. 2017. PMID: 28132690 Free PMC article.
-
Specific functions of Exostosin-like 3 (EXTL3) gene products.Cell Mol Biol Lett. 2020 Aug 20;25:39. doi: 10.1186/s11658-020-00231-y. eCollection 2020. Cell Mol Biol Lett. 2020. PMID: 32843889 Free PMC article. Review.
-
EXTL3-Associated Immunoskeletal Dysplasia with Neurodevelopmental Abnormalities: A Lethal Phenotype.Pediatr Allergy Immunol Pulmonol. 2023 Dec;36(4):147-149. doi: 10.1089/ped.2023.0079. Epub 2023 Nov 20. Pediatr Allergy Immunol Pulmonol. 2023. PMID: 38010729
-
Identification of biallelic EXTL3 mutations in a novel type of spondylo-epi-metaphyseal dysplasia.J Hum Genet. 2017 Aug;62(8):797-801. doi: 10.1038/jhg.2017.38. Epub 2017 Mar 23. J Hum Genet. 2017. PMID: 28331220 Free PMC article.
-
An ultra-rare case of immunoskeletal dysplasia with neurodevelopmental abnormalities in an Indian patient with homozygous c.953C > T variant in EXTL3 gene: a case report.BMC Pediatr. 2022 Feb 3;22(1):78. doi: 10.1186/s12887-022-03143-2. BMC Pediatr. 2022. PMID: 35114981 Free PMC article. Review.
Cited by
-
Supply chain logistics - the role of the Golgi complex in extracellular matrix production and maintenance.J Cell Sci. 2022 Jan 1;135(1):jcs258879. doi: 10.1242/jcs.258879. Epub 2022 Jan 13. J Cell Sci. 2022. PMID: 35023559 Free PMC article. Review.
-
Congenital Disorders of Deficiency in Glycosaminoglycan Biosynthesis.Front Genet. 2021 Sep 3;12:717535. doi: 10.3389/fgene.2021.717535. eCollection 2021. Front Genet. 2021. PMID: 34539746 Free PMC article. Review.
-
Complex Multisystem Phenotype With Immunodeficiency Associated With NBAS Mutations: Reports of Three Patients and Review of the Literature.Front Pediatr. 2020 Sep 15;8:577. doi: 10.3389/fped.2020.00577. eCollection 2020. Front Pediatr. 2020. PMID: 33042920 Free PMC article.
-
Investigation of the causal etiology in a patient with T-B+NK+ immunodeficiency.Front Immunol. 2022 Jul 29;13:928252. doi: 10.3389/fimmu.2022.928252. eCollection 2022. Front Immunol. 2022. PMID: 35967429 Free PMC article.
-
SLC10A7, an orphan member of the SLC10 family involved in congenital disorders of glycosylation.Hum Genet. 2022 Jul;141(7):1287-1298. doi: 10.1007/s00439-021-02420-x. Epub 2022 Jan 8. Hum Genet. 2022. PMID: 34999954
References
-
- Acquatella-Tran Van Ba I., Marchal S., François F., Silhol M., Lleres C., Michel B., Benyamin Y., Verdier J.M., Trousse F., and Marcilhac A.. 2012. Regenerating islet-derived 1α (Reg-1α) protein is new neuronal secreted factor that stimulates neurite outgrowth via exostosin Tumor-like 3 (EXTL3) receptor. J. Biol. Chem. 287:4726–4739. 10.1074/jbc.M111.260349 - DOI - PMC - PubMed
Publication types
MeSH terms
Substances
Grants and funding
LinkOut - more resources
Full Text Sources
Other Literature Sources
Medical
Molecular Biology Databases
Miscellaneous