The Central Role of Amino Acids in Cancer Redox Homeostasis: Vulnerability Points of the Cancer Redox Code
- PMID: 29312889
- PMCID: PMC5742588
- DOI: 10.3389/fonc.2017.00319
The Central Role of Amino Acids in Cancer Redox Homeostasis: Vulnerability Points of the Cancer Redox Code
Abstract
A fine balance in reactive oxygen species (ROS) production and removal is of utmost importance for homeostasis of all cells and especially in highly proliferating cells that encounter increased ROS production due to enhanced metabolism. Consequently, increased production of these highly reactive molecules requires coupling with increased antioxidant defense production within cells. This coupling is observed in cancer cells that allocate significant energy reserves to maintain their intracellular redox balance. Glutathione (GSH), as a first line of defense, represents the most important, non-enzymatic antioxidant component together with the NADPH/NADP+ couple, which ensures the maintenance of the pool of reduced GSH. In this review, the central role of amino acids (AAs) in the maintenance of redox homeostasis in cancer, through GSH synthesis (cysteine, glutamate, and glycine), and nicotinamide adenine dinucleotide (phosphate) production (serine, and glutamine/glutamate) are illustrated. Special emphasis is placed on the importance of AA transporters known to be upregulated in cancers (such as system xc-light chain and alanine-serine-cysteine transporter 2) in the maintenance of AA homeostasis, and thus indirectly, the redox homeostasis of cancer cells. The role of the ROS varies (often described as a "two-edged sword") during the processes of carcinogenesis, metastasis, and cancer treatment. Therefore, the context-dependent role of specific AAs in the initiation, progression, and dissemination of cancer, as well as in the redox-dependent sensitivity/resistance of the neoplastic cells to chemotherapy are highlighted.
Keywords: NADPH/NADP+; amino acids; cancer; glutathione; redox homeostasis.
Figures
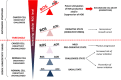
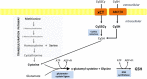
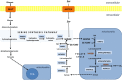
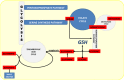
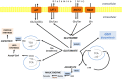
Similar articles
-
Role of glutamate transporters in redox homeostasis of the brain.Neurochem Int. 2014 Jul;73:181-91. doi: 10.1016/j.neuint.2014.01.001. Epub 2014 Jan 10. Neurochem Int. 2014. PMID: 24418113 Free PMC article.
-
A spontaneous mutation in the nicotinamide nucleotide transhydrogenase gene of C57BL/6J mice results in mitochondrial redox abnormalities.Free Radic Biol Med. 2013 Oct;63:446-56. doi: 10.1016/j.freeradbiomed.2013.05.049. Epub 2013 Jun 7. Free Radic Biol Med. 2013. PMID: 23747984
-
Pyridine nucleotide cycling and control of intracellular redox state in relation to poly (ADP-ribose) polymerase activity and nuclear localization of glutathione during exponential growth of Arabidopsis cells in culture.Mol Plant. 2009 May;2(3):442-56. doi: 10.1093/mp/ssp008. Epub 2009 Mar 23. Mol Plant. 2009. PMID: 19825628
-
Protein S-glutathionlyation links energy metabolism to redox signaling in mitochondria.Redox Biol. 2016 Aug;8:110-8. doi: 10.1016/j.redox.2015.12.010. Epub 2015 Dec 31. Redox Biol. 2016. PMID: 26773874 Free PMC article. Review.
-
Redox-Mediated Mechanism of Chemoresistance in Cancer Cells.Antioxidants (Basel). 2019 Oct 10;8(10):471. doi: 10.3390/antiox8100471. Antioxidants (Basel). 2019. PMID: 31658599 Free PMC article. Review.
Cited by
-
Diverse Stakeholders of Tumor Metabolism: An Appraisal of the Emerging Approach of Multifaceted Metabolic _targeting by 3-Bromopyruvate.Front Pharmacol. 2019 Jul 4;10:728. doi: 10.3389/fphar.2019.00728. eCollection 2019. Front Pharmacol. 2019. PMID: 31333455 Free PMC article. Review.
-
Free Amino Acid Alterations in Patients with Gynecological and Breast Cancer: A Review.Pharmaceuticals (Basel). 2021 Jul 27;14(8):731. doi: 10.3390/ph14080731. Pharmaceuticals (Basel). 2021. PMID: 34451829 Free PMC article. Review.
-
P-Glycoprotein: One Mechanism, Many Tasks and the Consequences for Pharmacotherapy of Cancers.Front Oncol. 2020 Oct 26;10:576559. doi: 10.3389/fonc.2020.576559. eCollection 2020. Front Oncol. 2020. PMID: 33194688 Free PMC article. Review.
-
Metabolic Adaptations in an Endocrine-Related Breast Cancer Mouse Model Unveil Potential Markers of Tumor Response to Hormonal Therapy.Front Oncol. 2022 Mar 1;12:786931. doi: 10.3389/fonc.2022.786931. eCollection 2022. Front Oncol. 2022. PMID: 35299741 Free PMC article.
-
Metabolomics of a cell line-derived xenograft model reveals circulating metabolic signatures for malignant mesothelioma.PeerJ. 2022 Jan 4;10:e12568. doi: 10.7717/peerj.12568. eCollection 2022. PeerJ. 2022. PMID: 35036082 Free PMC article.
References
Publication types
LinkOut - more resources
Full Text Sources
Other Literature Sources