A quantitative PCR-based assay reveals that nucleotide excision repair plays a predominant role in the removal of DNA-protein crosslinks from plasmids transfected into mammalian cells
- PMID: 29413806
- PMCID: PMC5811311
- DOI: 10.1016/j.dnarep.2018.01.004
A quantitative PCR-based assay reveals that nucleotide excision repair plays a predominant role in the removal of DNA-protein crosslinks from plasmids transfected into mammalian cells
Abstract
DNA-protein crosslinks (DPCs) are complex DNA lesions that induce mutagenesis and cell death. DPCs are created by common antitumor drugs, reactive oxygen species, and endogenous aldehydes. Since these agents create other types of DNA damage in addition to DPCs, identification of the mechanisms of DPC repair is challenging. In this study, we created plasmid substrates containing site-specific DPC lesions, as well as plasmids harboring lesions that are selectively repaired by the base excision or nucleotide excision repair (NER) pathways. These substrates were transfected into mammalian cells and a quantitative real-time PCR assay employed to study their repair. This assay revealed that DPC lesions were rapidly repaired in wild-type human and Chinese hamster derived cells, as were plasmids harboring an oxoguanine residue (base excision repair substrate) or cholesterol lesion (NER substrate). Interestingly, the DPC substrate was repaired in human cells nearly three times as efficiently as in Chinese hamster cells (>75% vs ∼25% repair at 8 h post-transfection), while there was no significant species-specific difference in the efficiency with which the cholesterol lesion was repaired (∼60% repair). Experiments revealed that both human and hamster cells deficient in NER due to mutations in the xeroderma pigmentosum A or D genes were five to ten-fold less able to repair the cholesterol and DPC lesions than were wild-type control clones, and that both the global genome and transcription-coupled sub-pathways of NER were capable of repairing DPCs. In addition, analyses using this PCR-based assay revealed that a 4 kDa peptide DNA crosslink was repaired nearly twice as efficiently as was a ∼38 kDa DPC, suggesting that proteolytic degradation of crosslinked proteins occurs during DPC repair. These results highlight the utility of this PCR-based assay to study DNA repair and indicate that the NER machinery rapidly and efficiently repairs plasmid DPC lesions in mammalian cells.
Keywords: DNA-protein crosslinks; Human oxoguanine glycosylase 1; Nucleotide excision repair; qPCR.
Copyright © 2018 The Authors. Published by Elsevier B.V. All rights reserved.
Conflict of interest statement
Figures
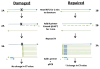
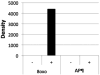
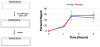
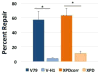
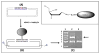
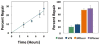
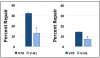
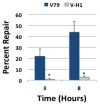
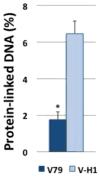
Similar articles
-
Loss of DNA-protein crosslinks from formaldehyde-exposed cells occurs through spontaneous hydrolysis and an active repair process linked to proteosome function.Carcinogenesis. 2000 Aug;21(8):1573-80. Carcinogenesis. 2000. PMID: 10910961
-
Nucleotide excision repair functions in the removal of chromium-induced DNA damage in mammalian cells.Mol Cell Biochem. 2005 Nov;279(1-2):85-95. doi: 10.1007/s11010-005-8225-0. Mol Cell Biochem. 2005. PMID: 16283517
-
Ubiquitin signaling and the proteasome drive human DNA-protein crosslink repair.Nucleic Acids Res. 2023 Dec 11;51(22):12174-12184. doi: 10.1093/nar/gkad860. Nucleic Acids Res. 2023. PMID: 37843153 Free PMC article.
-
Formation and repair of DNA-protein crosslink damage.Sci China Life Sci. 2017 Oct;60(10):1065-1076. doi: 10.1007/s11427-017-9183-4. Epub 2017 Oct 30. Sci China Life Sci. 2017. PMID: 29098631 Free PMC article. Review.
-
Radiation-induced DNA-protein cross-links: Mechanisms and biological significance.Free Radic Biol Med. 2017 Jun;107:136-145. doi: 10.1016/j.freeradbiomed.2016.11.041. Epub 2016 Nov 25. Free Radic Biol Med. 2017. PMID: 27894771 Review.
Cited by
-
Transcriptional Bypass of DNA-Protein and DNA-Peptide Conjugates by T7 RNA Polymerase.ACS Chem Biol. 2019 Dec 20;14(12):2564-2575. doi: 10.1021/acschembio.9b00365. Epub 2019 Oct 21. ACS Chem Biol. 2019. PMID: 31573793 Free PMC article.
-
Enzymatic bypass of an N6-deoxyadenosine DNA-ethylene dibromide-peptide cross-link by translesion DNA polymerases.J Biol Chem. 2021 Jan-Jun;296:100444. doi: 10.1016/j.jbc.2021.100444. Epub 2021 Feb 20. J Biol Chem. 2021. PMID: 33617883 Free PMC article.
-
5-Formylcytosine-induced DNA-peptide cross-links reduce transcription efficiency, but do not cause transcription errors in human cells.J Biol Chem. 2019 Nov 29;294(48):18387-18397. doi: 10.1074/jbc.RA119.009834. Epub 2019 Oct 9. J Biol Chem. 2019. PMID: 31597704 Free PMC article.
-
Tyrosyl-DNA phosphodiesterase 2 (Tdp2) repairs DNA-protein crosslinks and protects against double strand breaks in vivo.Front Cell Dev Biol. 2024 Aug 20;12:1394531. doi: 10.3389/fcell.2024.1394531. eCollection 2024. Front Cell Dev Biol. 2024. PMID: 39228401 Free PMC article.
-
DNA-protein crosslinks are repaired via homologous recombination in mammalian mitochondria.DNA Repair (Amst). 2021 Jan;97:103026. doi: 10.1016/j.dnarep.2020.103026. Epub 2020 Nov 25. DNA Repair (Amst). 2021. PMID: 33316746 Free PMC article.
References
-
- Vaz B, Popovic M, Ramadan K. DNA-Protein Crosslink Proteolysis Repair. Trends Biochem Sci. 2017;42:483–495. - PubMed
Publication types
MeSH terms
Substances
Grants and funding
LinkOut - more resources
Full Text Sources
Other Literature Sources