Progress of Inertial Microfluidics in Principle and Application
- PMID: 29857563
- PMCID: PMC6021949
- DOI: 10.3390/s18061762
Progress of Inertial Microfluidics in Principle and Application
Abstract
Inertial microfluidics has become a popular topic in microfluidics research for its good performance in particle manipulation and its advantages of simple structure, high throughput, and freedom from an external field. Compared with traditional microfluidic devices, the flow field in inertial microfluidics is between Stokes state and turbulence, whereas the flow is still regarded as laminar. However, many mechanical effects induced by the inertial effect are difficult to observe in traditional microfluidics, making particle motion analysis in inertial microfluidics more complicated. In recent years, the inertial migration effect in straight and curved channels has been explored theoretically and experimentally to realize on-chip manipulation with extensive applications from the ordinary manipulation of particles to biochemical analysis. In this review, the latest theoretical achievements and force analyses of inertial microfluidics and its development process are introduced, and its applications in circulating tumor cells, exosomes, DNA, and other biological particles are summarized. Finally, the future development of inertial microfluidics is discussed. Owing to its special advantages in particle manipulation, inertial microfluidics will play a more important role in integrated biochips and biomolecule analysis.
Keywords: Dean vortex; inertial microfluidics; lab-on-a-chip; particle manipulation.
Conflict of interest statement
The authors declare no conflict of interest.
Figures
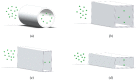
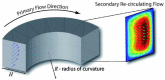
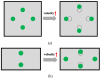
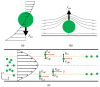
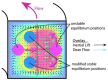
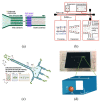
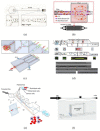
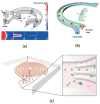
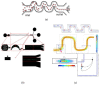
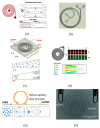
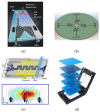
Similar articles
-
Inertial microfluidics: Recent advances.Electrophoresis. 2020 Dec;41(24):2166-2187. doi: 10.1002/elps.202000134. Epub 2020 Oct 21. Electrophoresis. 2020. PMID: 33027533 Review.
-
Channel innovations for inertial microfluidics.Lab Chip. 2020 Oct 7;20(19):3485-3502. doi: 10.1039/d0lc00714e. Epub 2020 Sep 10. Lab Chip. 2020. PMID: 32910129
-
A Review of Secondary Flow in Inertial Microfluidics.Micromachines (Basel). 2020 Apr 28;11(5):461. doi: 10.3390/mi11050461. Micromachines (Basel). 2020. PMID: 32354106 Free PMC article. Review.
-
Recent progress of inertial microfluidic-based cell separation.Analyst. 2021 Nov 22;146(23):7070-7086. doi: 10.1039/d1an01160j. Analyst. 2021. PMID: 34761757 Review.
-
Oscillatory inertial focusing in infinite microchannels.Proc Natl Acad Sci U S A. 2018 Jul 24;115(30):7682-7687. doi: 10.1073/pnas.1721420115. Epub 2018 Jul 10. Proc Natl Acad Sci U S A. 2018. PMID: 29991599 Free PMC article.
Cited by
-
Urine biopsy technologies: Cancer and beyond.Theranostics. 2020 Jun 22;10(17):7872-7888. doi: 10.7150/thno.44634. eCollection 2020. Theranostics. 2020. PMID: 32685026 Free PMC article. Review.
-
3D Printing of Inertial Microfluidic Devices.Sci Rep. 2020 Apr 3;10(1):5929. doi: 10.1038/s41598-020-62569-9. Sci Rep. 2020. PMID: 32246111 Free PMC article.
-
Establishment of a Perfusion Process with Antibody-Producing CHO Cells Using a 3D-Printed Microfluidic Spiral Separator with Web-Based Flow Control.Bioengineering (Basel). 2023 May 28;10(6):656. doi: 10.3390/bioengineering10060656. Bioengineering (Basel). 2023. PMID: 37370588 Free PMC article.
-
Particle Focusing under Newtonian and Viscoelastic Flow in a Straight Rhombic Microchannel.Micromachines (Basel). 2020 Nov 11;11(11):998. doi: 10.3390/mi11110998. Micromachines (Basel). 2020. PMID: 33187390 Free PMC article.
-
Low-cost inertial microfluidic device for microparticle separation: A laser-Ablated PMMA lab-on-a-chip approach without a cleanroom.HardwareX. 2023 Nov 11;16:e00493. doi: 10.1016/j.ohx.2023.e00493. eCollection 2023 Dec. HardwareX. 2023. PMID: 38045919 Free PMC article.
References
Publication types
LinkOut - more resources
Full Text Sources
Other Literature Sources
Miscellaneous