SSPIM: a beam shaping toolbox for structured selective plane illumination microscopy
- PMID: 29968787
- PMCID: PMC6030192
- DOI: 10.1038/s41598-018-28389-8
SSPIM: a beam shaping toolbox for structured selective plane illumination microscopy
Abstract
Selective plane illumination microscopy (SPIM) represents a preferred method in dynamic tissue imaging, because it combines high spatiotemporal resolution with low phototoxicity. The OpenSPIM system was developed to provide an accessible and flexible microscope set-up for non-specialist users. Here, we report Structured SPIM (SSPIM), which offers an open-source, user-friendly and compact toolbox for beam shaping to be applied within the OpenSPIM platform. SSPIM is able to generate digital patterns for a wide range of illumination beams including static and spherical Gaussian beams, Bessel beams and Airy beams by controlling the pattern of a Spatial Light Modulator (SLM). In addition, SSPIM can produce patterns for structured illumination including incoherent and coherent array beams and tiling for all types of the supported beams. We describe the workflow of the toolbox and demonstrate its application by comparing experimental data with simulation results for a wide range of illumination beams. Finally, the capability of SSPIM is investigated by 3D imaging of Drosophila embryos using scanned Gaussian, Bessel and array beams. SSPIM provides an accessible toolbox to generate and optimize the desired beam patterns and helps adapting the OpenSPIM system towards a wider range of biological samples.
Conflict of interest statement
The authors declare no competing interests.
Figures
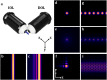
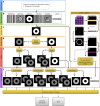
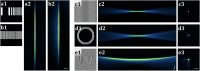
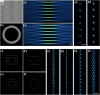
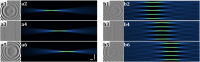
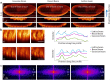
Similar articles
-
Multi-purpose SLM-light-sheet microscope.Biomed Opt Express. 2018 Oct 12;9(11):5419-5436. doi: 10.1364/BOE.9.005419. eCollection 2018 Nov 1. Biomed Opt Express. 2018. PMID: 30460137 Free PMC article.
-
Light-sheet microscopy for everyone? Experience of building an OpenSPIM to study flatworm development.BMC Dev Biol. 2016 Jun 30;16(1):22. doi: 10.1186/s12861-016-0122-0. BMC Dev Biol. 2016. PMID: 27363495 Free PMC article.
-
A compact Airy beam light sheet microscope with a tilted cylindrical lens.Biomed Opt Express. 2014 Sep 5;5(10):3434-42. doi: 10.1364/BOE.5.003434. eCollection 2014 Oct 1. Biomed Opt Express. 2014. PMID: 25360362 Free PMC article.
-
Sample Preparation and Mounting of Drosophila Embryos for Multiview Light Sheet Microscopy.Methods Mol Biol. 2016;1478:189-202. doi: 10.1007/978-1-4939-6371-3_10. Methods Mol Biol. 2016. PMID: 27730582 Review.
-
Light sheet microscopes: Novel imaging toolbox for visualizing life's processes.Int J Biochem Cell Biol. 2016 Nov;80:119-123. doi: 10.1016/j.biocel.2016.10.002. Epub 2016 Oct 8. Int J Biochem Cell Biol. 2016. PMID: 27725275 Review.
Cited by
-
Bessel light beam for a surgical laser focusing telescope-a novel approach.Lasers Med Sci. 2024 Jan 17;39(1):33. doi: 10.1007/s10103-023-03968-y. Lasers Med Sci. 2024. PMID: 38231430
-
Multi-purpose SLM-light-sheet microscope.Biomed Opt Express. 2018 Oct 12;9(11):5419-5436. doi: 10.1364/BOE.9.005419. eCollection 2018 Nov 1. Biomed Opt Express. 2018. PMID: 30460137 Free PMC article.
-
Multicolor iLIFE (m-iLIFE) volume cytometry for high-throughput imaging of multiple organelles.Sci Rep. 2024 Oct 11;14(1):23798. doi: 10.1038/s41598-024-73667-3. Sci Rep. 2024. PMID: 39394224 Free PMC article.
-
Bessel Beam: Significance and Applications-A Progressive Review.Micromachines (Basel). 2020 Nov 11;11(11):997. doi: 10.3390/mi11110997. Micromachines (Basel). 2020. PMID: 33187147 Free PMC article. Review.
-
Light sheet fluorescence microscopy for the investigation of blood-sucking arthropods dyed via artificial membrane feeding.Parasit Vectors. 2022 Feb 12;15(1):52. doi: 10.1186/s13071-022-05157-2. Parasit Vectors. 2022. PMID: 35151358 Free PMC article.
References
-
- Fahrbach FO, Simon P, Rohrbach A. Microscopy with self-reconstructing beams. Nat. Photonics. 2010;4:780–785. doi: 10.1038/nphoton.2010.204. - DOI
Publication types
LinkOut - more resources
Full Text Sources
Other Literature Sources
Molecular Biology Databases