Medicinal Leech CNS as a Model for Exosome Studies in the Crosstalk between Microglia and Neurons
- PMID: 30572617
- PMCID: PMC6321190
- DOI: 10.3390/ijms19124124
Medicinal Leech CNS as a Model for Exosome Studies in the Crosstalk between Microglia and Neurons
Abstract
In healthy or pathological brains, the neuroinflammatory state is supported by a strong communication involving microglia and neurons. Recent studies indicate that extracellular vesicles (EVs), including exosomes and microvesicles, play a key role in the physiological interactions between cells allowing central nervous system (CNS) development and/or integrity. The present report used medicinal leech CNS to investigate microglia/neuron crosstalk from ex vivo approaches as well as primary cultures. The results demonstrated a large production of exosomes from microglia. Their incubation to primary neuronal cultures showed a strong interaction with neurites. In addition, neurite outgrowth assays demonstrated microglia exosomes to exhibit significant neurotrophic activities using at least a Transforming Growth Factor beta (TGF-β) family member, called nGDF (nervous Growth/Differentiation Factor). Of interest, the results also showed an EV-mediated dialog between leech microglia and rat cells highlighting this communication to be more a matter of molecules than of species. Taken together, the present report brings a new insight into the microglia/neuron crosstalk in CNS and would help deciphering the molecular evolution of such a cell communication in brain.
Keywords: exosomes; leech; microglia; neurite outgrowth.
Conflict of interest statement
The authors declare no conflict of interest.
Figures
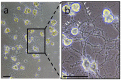
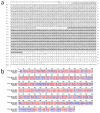
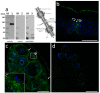
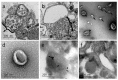
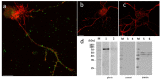
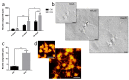
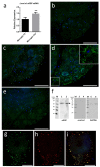
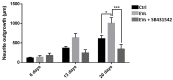
Similar articles
-
Isolation of microglia-derived extracellular vesicles: towards miRNA signatures and neuroprotection.J Nanobiotechnology. 2019 Dec 4;17(1):119. doi: 10.1186/s12951-019-0551-6. J Nanobiotechnology. 2019. PMID: 31801555 Free PMC article.
-
Microbial challenge promotes the regenerative process of the injured central nervous system of the medicinal leech by inducing the synthesis of antimicrobial peptides in neurons and microglia.J Immunol. 2008 Jul 15;181(2):1083-95. doi: 10.4049/jimmunol.181.2.1083. J Immunol. 2008. PMID: 18606660 Free PMC article.
-
The leech nervous system: a valuable model to study the microglia involvement in regenerative processes.Clin Dev Immunol. 2013;2013:274019. doi: 10.1155/2013/274019. Epub 2013 Jun 26. Clin Dev Immunol. 2013. PMID: 23878582 Free PMC article. Review.
-
Evidence for a novel chemotactic C1q domain-containing factor in the leech nerve cord.Mol Immunol. 2009 Feb;46(4):523-31. doi: 10.1016/j.molimm.2008.07.026. Epub 2008 Oct 25. Mol Immunol. 2009. PMID: 18952286
-
The Messenger Apps of the cell: Extracellular Vesicles as Regulatory Messengers of Microglial Function in the CNS.J Neuroimmune Pharmacol. 2020 Sep;15(3):473-486. doi: 10.1007/s11481-020-09916-9. Epub 2020 Apr 27. J Neuroimmune Pharmacol. 2020. PMID: 32337651 Free PMC article. Review.
Cited by
-
The multiple faces of extracellular vesicles released by microglia: Where are we 10 years after?Front Cell Neurosci. 2022 Sep 13;16:984690. doi: 10.3389/fncel.2022.984690. eCollection 2022. Front Cell Neurosci. 2022. PMID: 36176630 Free PMC article. Review.
-
ALK4/5-dependent TGF-β signaling contributes to the crosstalk between neurons and microglia following axonal lesion.Sci Rep. 2019 May 3;9(1):6896. doi: 10.1038/s41598-019-43328-x. Sci Rep. 2019. PMID: 31053759 Free PMC article.
-
Alternative proteins are functional regulators in cell reprogramming by PKA activation.Nucleic Acids Res. 2020 Aug 20;48(14):7864-7882. doi: 10.1093/nar/gkaa277. Nucleic Acids Res. 2020. PMID: 32324228 Free PMC article.
-
Current Knowledge of Microglia in Traumatic Spinal Cord Injury.Front Neurol. 2022 Jan 11;12:796704. doi: 10.3389/fneur.2021.796704. eCollection 2021. Front Neurol. 2022. PMID: 35087472 Free PMC article. Review.
-
Exosomes in Cerebral Ischemia-Reperfusion Injury: Current Perspectives and Future Challenges.Brain Sci. 2022 Dec 2;12(12):1657. doi: 10.3390/brainsci12121657. Brain Sci. 2022. PMID: 36552117 Free PMC article. Review.
References
-
- Schafer D.P., Lehrman E.K., Kautzman A.G., Koyama R., Mardinly A.R., Yamasaki R., Ransohoff R.M., Greenberg M.E., Barres B.A., Stevens B. Microglia Sculpt Postnatal Neural Circuits in an Activity and Complement-Dependent Manner. Neuron. 2012;74:691–705. doi: 10.1016/j.neuron.2012.03.026. - DOI - PMC - PubMed
-
- Zhan Y., Paolicelli R.C., Sforazzini F., Weinhard L., Bolasco G., Pagani F., Vyssotski A.L., Bifone A., Gozzi A., Ragozzino D., et al. Deficient Neuron-Microglia Signaling Results in Impaired Functional Brain Connectivity and Social Behavior. Nat. Neurosci. 2014;17:400–406. doi: 10.1038/nn.3641. - DOI - PubMed
MeSH terms
Substances
LinkOut - more resources
Full Text Sources