Protective effects of Bacillus probiotics against high-fat diet-induced metabolic disorders in mice
- PMID: 30596786
- PMCID: PMC6312313
- DOI: 10.1371/journal.pone.0210120
Protective effects of Bacillus probiotics against high-fat diet-induced metabolic disorders in mice
Abstract
Recently, modulation of gut microbiota by probiotics treatment has been emerged as a promising strategy for treatment of metabolic disorders. Apart from lactic acid bacteria, Bacillus species (Bacillus spp.) have also been paid attention as potential probiotics, but nevertheless, the molecular mechanisms for their protective effect against metabolic dysfunction remain to be elucidated. In this study, we demonstrate that a probiotic mixture composed of 5 different Bacillus spp. protects mice from high-fat diet (HFD)-induced obesity, insulin resistance and non-alcoholic fatty liver disease (NAFLD). Probiotic Bacillus treatment substantially attenuated body weight gain and enhanced glucose tolerance by sensitizing insulin action in skeletal muscle and epididymal adipose tissue (EAT) of HFD-fed mice. Bacillus-treated HFD-fed mice also exhibited significantly suppressed chronic inflammation in the liver, EAT and skeletal muscle, which was observed to be associated with reduced HFD-induced intestinal permeability and enhanced adiponectin production. Additionally, Bacillus treatment significantly reversed HFD-induced hepatic steatosis. In Bacillus-treated mice, hepatic expression of lipid oxidative genes was significantly increased, and lipid accumulation in subcutaneous and mesenteric adipose tissues were significantly decreased, commensurate with down-regulated expression of genes involved in lipid uptake and lipogenesis. Although, in Bacillus-treated mice, significant alterations in gut microbiota composition was not observed, the enhanced expression of tight junction-associated proteins showed a possibility of improving gut barrier function by Bacillus treatment. Our findings provide possible explanations how Bacillus probiotics protect diet-induced obese mice against metabolic disorders, identifying the treatment of probiotic Bacillus as a potential therapeutic approach.
Conflict of interest statement
Yosep Ji and Wilhelm Holzapfel, who are board members of Holzapfel Effective Microbes (HEM), declare conflict of interest as they have collaborated in gut microbiota analysis and this study was partially funded by HEM. The other authors declare no conflict of interest. This does not alter our adherence to PLOS ONE policies on sharing data and materials.
Figures
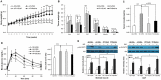
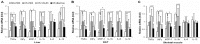
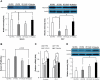
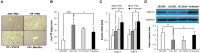
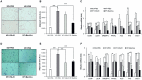
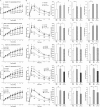
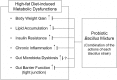
Similar articles
-
Long-term fermented soybean paste improves metabolic parameters associated with non-alcoholic fatty liver disease and insulin resistance in high-fat diet-induced obese mice.Biochem Biophys Res Commun. 2018 Jan 8;495(2):1744-1751. doi: 10.1016/j.bbrc.2017.12.003. Epub 2017 Dec 6. Biochem Biophys Res Commun. 2018. PMID: 29222051
-
Improvement in glucose tolerance and insulin sensitivity by probiotic strains of Indian gut origin in high-fat diet-fed C57BL/6J mice.Eur J Nutr. 2018 Feb;57(1):279-295. doi: 10.1007/s00394-016-1317-7. Epub 2016 Oct 18. Eur J Nutr. 2018. PMID: 27757592
-
Anti-obesogenic effects of WY14643 (PPAR-alpha agonist): Hepatic mitochondrial enhancement and suppressed lipogenic pathway in diet-induced obese mice.Biochimie. 2017 Sep;140:106-116. doi: 10.1016/j.biochi.2017.07.003. Epub 2017 Jul 12. Biochimie. 2017. PMID: 28711683
-
Usefulness of Probiotics in the Management of NAFLD: Evidence and Involved Mechanisms of Action from Preclinical and Human Models.Int J Mol Sci. 2022 Mar 15;23(6):3167. doi: 10.3390/ijms23063167. Int J Mol Sci. 2022. PMID: 35328587 Free PMC article. Review.
-
Protective and ameliorating effects of probiotics against diet-induced obesity: A review.Food Res Int. 2021 Sep;147:110490. doi: 10.1016/j.foodres.2021.110490. Epub 2021 Jun 2. Food Res Int. 2021. PMID: 34399486 Review.
Cited by
-
Pretreatment With Bacillus cereus Preserves Against D-Galactosamine-Induced Liver Injury in a Rat Model.Front Microbiol. 2019 Jul 31;10:1751. doi: 10.3389/fmicb.2019.01751. eCollection 2019. Front Microbiol. 2019. PMID: 31417535 Free PMC article.
-
Hypocholesterolemic potential of Bacillus amyloliquefaciens KAVK1 modulates lipid accumulation on 3T3-L1 adipose cells and high fat diet-induced obese rat model.World J Microbiol Biotechnol. 2024 May 17;40(7):206. doi: 10.1007/s11274-024-04016-9. World J Microbiol Biotechnol. 2024. PMID: 38755297
-
Metagenomic Analysis Reveals A Gut Microbiota Structure and Function Alteration between Healthy and Diarrheic Juvenile Yaks.Animals (Basel). 2024 Apr 14;14(8):1181. doi: 10.3390/ani14081181. Animals (Basel). 2024. PMID: 38672329 Free PMC article.
-
Probiotics for treatment of nonalcoholic fatty liver disease: It is worth a try.Clin Mol Hepatol. 2021 Jan;27(1):83-86. doi: 10.3350/cmh.2020.0298. Epub 2020 Dec 3. Clin Mol Hepatol. 2021. PMID: 33317240 Free PMC article. No abstract available.
-
Modelling a Western Lifestyle in Mice: A Novel Approach to Eradicating Aerobic Spore-Forming Bacteria from the Colonic Microbiome and Assessing Long-Term Clinical Outcomes.Biomedicines. 2024 Oct 7;12(10):2274. doi: 10.3390/biomedicines12102274. Biomedicines. 2024. PMID: 39457587 Free PMC article.
References
Publication types
MeSH terms
Substances
Grants and funding
LinkOut - more resources
Full Text Sources
Other Literature Sources
Medical
Miscellaneous