Glutamate Within the Marmoset Anterior Hippocampus Interacts with Area 25 to Regulate the Behavioral and Cardiovascular Correlates of High-Trait Anxiety
- PMID: 30718320
- PMCID: PMC6468106
- DOI: 10.1523/JNEUROSCI.2451-18.2018
Glutamate Within the Marmoset Anterior Hippocampus Interacts with Area 25 to Regulate the Behavioral and Cardiovascular Correlates of High-Trait Anxiety
Abstract
High-trait anxiety is a risk factor for the development of affective disorders and has been associated with decreased cardiovascular and behavioral responsivity to acute stressors in humans that may increase the risk of developing cardiovascular disease. Although human neuroimaging studies of high-trait anxiety reveals dysregulation in primate cingulate areas 25 and 32 and the anterior hippocampus (aHipp) and rodent studies reveal the importance of aHipp glutamatergic hypofunction, the causal involvement of aHipp glutamate and its interaction with these areas in the primate brain is unknown. Accordingly, we correlated marmoset trait anxiety scores to their postmortem aHipp glutamate levels and showed that low glutamate in the right aHipp is associated with high-trait anxiety in marmosets. Moreover, pharmacologically increasing aHipp glutamate reduced anxiety levels in highly anxious marmosets in two uncertainty-based tests of anxiety: exposure to a human intruder with uncertain intent and unpredictable loud noise. In the human intruder test, increasing aHipp glutamate decreased anxiety by increasing approach to the intruder. In the unpredictable threat test, animals showed blunted behavioral and cardiovascular responsivity after control infusions, which was normalized by increasing aHipp glutamate. However, this aHipp-mediated anxiolytic effect was blocked by simultaneous pharmacological inactivation of area 25, but not area 32, areas which when inactivated independently reduced and had no effect on anxiety, respectively. These findings provide causal evidence in male and female primates that aHipp glutamatergic hypofunction and its regulation by area 25 contribute to the behavioral and cardiovascular symptoms of endogenous high-trait anxiety.SIGNIFICANCE STATEMENT High-trait anxiety predisposes sufferers to the development of anxiety and depression. Although neuroimaging of these disorders and rodent modeling implicate dysregulation in hippocampal glutamate and the subgenual/perigenual cingulate cortices (areas 25/32), the causal involvement of these structures in endogenous high-trait anxiety and their interaction are unknown. Here, we demonstrate that increased trait anxiety in marmoset monkeys correlates with reduced hippocampal glutamate and that increasing hippocampal glutamate release in high-trait-anxious monkeys normalizes the aberrant behavioral and cardiovascular responsivity to potential threats. This normalization was blocked by simultaneous inactivation of area 25, but not area 32. These findings provide casual evidence in primates that hippocampal glutamatergic hypofunction regulates endogenous high-trait anxiety and the hippocampal-area 25 circuit is a potential therapeutic _target.
Keywords: anxiety; area 25; cardiovascular; glutamate; hippocampus; marmoset.
Copyright © 2019 Zeredo et al.
Conflict of interest statement
The authors declare no competing financial interests.
Figures
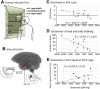
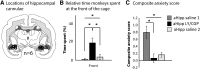
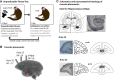
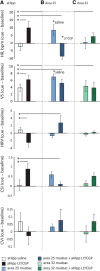
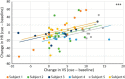
Similar articles
-
Hippocampal Interaction With Area 25, but not Area 32, Regulates Marmoset Approach-Avoidance Behavior.Cereb Cortex. 2019 Dec 17;29(11):4818-4830. doi: 10.1093/cercor/bhz015. Cereb Cortex. 2019. PMID: 30796800 Free PMC article.
-
Anxiety-like behavior of prenatally stressed rats is associated with a selective reduction of glutamate release in the ventral hippocampus.J Neurosci. 2012 Nov 28;32(48):17143-54. doi: 10.1523/JNEUROSCI.1040-12.2012. J Neurosci. 2012. PMID: 23197707 Free PMC article.
-
Trait Anxiety Mediated by Amygdala Serotonin Transporter in the Common Marmoset.J Neurosci. 2020 Jun 10;40(24):4739-4749. doi: 10.1523/JNEUROSCI.2930-19.2020. Epub 2020 May 11. J Neurosci. 2020. PMID: 32393533 Free PMC article.
-
Behavioral and serotonergic consequences of decreasing or increasing hippocampus brain-derived neurotrophic factor protein levels in mice.Neuropharmacology. 2008 Nov;55(6):1006-14. doi: 10.1016/j.neuropharm.2008.08.001. Epub 2008 Aug 12. Neuropharmacology. 2008. PMID: 18761360 Review.
-
Subcallosal area 25: Its responsivity to the stress hormone cortisol and its opposing effects on appetitive motivation in marmosets.Neurobiol Stress. 2024 May 1;31:100637. doi: 10.1016/j.ynstr.2024.100637. eCollection 2024 Jul. Neurobiol Stress. 2024. PMID: 38741617 Free PMC article. Review.
Cited by
-
Serial Prefrontal Pathways Are Positioned to Balance Cognition and Emotion in Primates.J Neurosci. 2020 Oct 21;40(43):8306-8328. doi: 10.1523/JNEUROSCI.0860-20.2020. Epub 2020 Sep 28. J Neurosci. 2020. PMID: 32989097 Free PMC article.
-
A Focus on the Functions of Area 25.Brain Sci. 2019 Jun 3;9(6):129. doi: 10.3390/brainsci9060129. Brain Sci. 2019. PMID: 31163643 Free PMC article. Review.
-
Ventromedial prefrontal area 14 provides opposing regulation of threat and reward-elicited responses in the common marmoset.Proc Natl Acad Sci U S A. 2020 Oct 6;117(40):25116-25127. doi: 10.1073/pnas.2009657117. Epub 2020 Sep 21. Proc Natl Acad Sci U S A. 2020. PMID: 32958652 Free PMC article.
-
Understanding them to understand ourselves: The importance of NHP research for translational neuroscience.Curr Res Neurobiol. 2022 Aug 17;3:100049. doi: 10.1016/j.crneur.2022.100049. eCollection 2022. Curr Res Neurobiol. 2022. PMID: 36518342 Free PMC article.
-
Serum metabolomic abnormalities in survivors of non-severe COVID-19.Heliyon. 2022 Sep;8(9):e10473. doi: 10.1016/j.heliyon.2022.e10473. Epub 2022 Sep 1. Heliyon. 2022. PMID: 36065322 Free PMC article.
References
-
- Agustín-Pavón C, Braesicke K, Shiba Y, Santangelo AM, Mikheenko Y, Cockroft G, Asma F, Clarke H, Man MS, Roberts AC (2012) Lesions of ventrolateral prefrontal or anterior orbitofrontal cortex in primates heighten negative emotion. Biol Psychiatry 72:266–272. 10.1016/j.biopsych.2012.03.007 - DOI - PubMed
Publication types
MeSH terms
Substances
Grants and funding
LinkOut - more resources
Full Text Sources
Other Literature Sources
Medical