Engineered SUMO/protease system identifies Pdr6 as a bidirectional nuclear transport receptor
- PMID: 31023724
- PMCID: PMC6548132
- DOI: 10.1083/jcb.201812091
Engineered SUMO/protease system identifies Pdr6 as a bidirectional nuclear transport receptor
Abstract
Cleavage of affinity tags by specific proteases can be exploited for highly selective affinity chromatography. The SUMO/SENP1 system is the most efficient for such application but fails in eukaryotic expression because it cross-reacts with endogenous proteases. Using a novel selection system, we have evolved the SUMOEu/SENP1Eu pair to orthogonality with the yeast and animal enzymes. SUMOEu fusions therefore remain stable in eukaryotic cells. Likewise, overexpressing a SENP1Eu protease is nontoxic in yeast. We have used the SUMOEu system in an affinity-capture-proteolytic-release approach to identify interactors of the yeast importin Pdr6/Kap122. This revealed not only further nuclear import substrates such as Ubc9, but also Pil1, Lsp1, eIF5A, and eEF2 as RanGTP-dependent binders and thus as export cargoes. We confirmed that Pdr6 functions as an exportin in vivo and depletes eIF5A and eEF2 from cell nuclei. Thus, Pdr6 is a bidirectional nuclear transport receptor (i.e., a biportin) that shuttles distinct sets of cargoes in opposite directions.
© 2019 Vera Rodriguez et al.
Figures
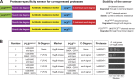
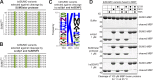
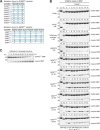
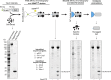
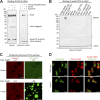
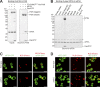
Similar articles
-
Structural basis for the nuclear import and export functions of the biportin Pdr6/Kap122.J Cell Biol. 2019 Jun 3;218(6):1839-1852. doi: 10.1083/jcb.201812093. Epub 2019 Apr 25. J Cell Biol. 2019. PMID: 31023722 Free PMC article.
-
A global S. cerevisiae small ubiquitin-related modifier (SUMO) system interactome.Mol Syst Biol. 2013 May 28;9:668. doi: 10.1038/msb.2013.23. Mol Syst Biol. 2013. PMID: 23712011 Free PMC article.
-
Essential role of nuclear localization for yeast Ulp2 SUMO protease function.Mol Biol Cell. 2009 Apr;20(8):2196-206. doi: 10.1091/mbc.e08-10-1090. Epub 2009 Feb 18. Mol Biol Cell. 2009. PMID: 19225149 Free PMC article.
-
SUMO-specific proteases: a twist in the tail.Trends Cell Biol. 2007 Aug;17(8):370-6. doi: 10.1016/j.tcb.2007.08.002. Epub 2007 Sep 4. Trends Cell Biol. 2007. PMID: 17768054 Review.
-
Function and regulation of SUMO proteases.Nat Rev Mol Cell Biol. 2012 Dec;13(12):755-66. doi: 10.1038/nrm3478. Nat Rev Mol Cell Biol. 2012. PMID: 23175280 Free PMC article. Review.
Cited by
-
Synapsin condensation controls synaptic vesicle sequestering and dynamics.Nat Commun. 2023 Oct 23;14(1):6730. doi: 10.1038/s41467-023-42372-6. Nat Commun. 2023. PMID: 37872159 Free PMC article.
-
A selectivity filter in the ER membrane protein complex limits protein misinsertion at the ER.J Cell Biol. 2023 Aug 7;222(8):e202212007. doi: 10.1083/jcb.202212007. Epub 2023 May 18. J Cell Biol. 2023. PMID: 37199759 Free PMC article.
-
PEX5 translocation into and out of peroxisomes drives matrix protein import.Mol Cell. 2022 Sep 1;82(17):3209-3225.e7. doi: 10.1016/j.molcel.2022.07.004. Epub 2022 Aug 4. Mol Cell. 2022. PMID: 35931083 Free PMC article.
-
Efficient in planta production of amidated antimicrobial peptides that are active against drug-resistant ESKAPE pathogens.Nat Commun. 2023 Mar 16;14(1):1464. doi: 10.1038/s41467-023-37003-z. Nat Commun. 2023. PMID: 36928189 Free PMC article.
-
Molecular basis of RanGTP-activated release of Histones H2A-H2B from Importin-9.Structure. 2023 Aug 3;31(8):903-911.e3. doi: 10.1016/j.str.2023.06.001. Epub 2023 Jun 27. Structure. 2023. PMID: 37379840 Free PMC article.
References
Publication types
MeSH terms
Substances
LinkOut - more resources
Full Text Sources
Other Literature Sources
Molecular Biology Databases
Research Materials
Miscellaneous