NAD+ precursor modulates post-ischemic mitochondrial fragmentation and reactive oxygen species generation via SIRT3 dependent mechanisms
- PMID: 31837320
- PMCID: PMC8328278
- DOI: 10.1016/j.expneurol.2019.113144
NAD+ precursor modulates post-ischemic mitochondrial fragmentation and reactive oxygen species generation via SIRT3 dependent mechanisms
Abstract
Global cerebral ischemia depletes brain tissue NAD+, an essential cofactor for mitochondrial and cellular metabolism, leading to bioenergetics failure and cell death. The post-ischemic NAD+ levels can be replenished by the administration of nicotinamide mononucleotide (NMN), which serves as a precursor for NAD+ synthesis. We have shown that NMN administration shows dramatic protection against ischemic brain damage and inhibits post-ischemic hippocampal mitochondrial fragmentation. To understand the mechanism of NMN-induced modulation of mitochondrial dynamics and neuroprotection we used our transgenic mouse models that express mitochondria _targeted yellow fluorescent protein in neurons (mito-eYFP) and mice that carry knockout of mitochondrial NAD+-dependent deacetylase sirt3 gene (SIRT3KO). Following ischemic insult, the mitochondrial NAD+ levels were depleted leading to an increase in mitochondrial protein acetylation, high reactive oxygen species (ROS) production, and excessive mitochondrial fragmentation. Administration of a single dose of NMN normalized hippocampal mitochondria NAD+ pools, protein acetylation, and ROS levels. These changes were dependent on SIRT3 activity, which was confirmed using SIRT3KO mice. Ischemia induced increase in acetylation of the key mitochondrial antioxidant enzyme, superoxide dismutase 2 (SOD2) that resulted in inhibition of its activity. This was reversed after NMN treatment followed by reduction of ROS generation and suppression of mitochondrial fragmentation. Specifically, we found that the interaction of mitochondrial fission protein, pDrp1(S616), with neuronal mitochondria was inhibited in NMN treated ischemic mice. Our data thus provide a novel link between mitochondrial NAD+ metabolism, ROS production, and mitochondrial fragmentation. Using NMN to _target these mechanisms could represent a new therapeutic approach for treatment of acute brain injury and neurodegenerative diseases.
Keywords: Acetylation; Free radicals; Global cerebral ischemia; Mitochondria; Mitochondrial dynamics; NAD(+).
Copyright © 2019 Elsevier Inc. All rights reserved.
Figures
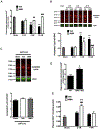
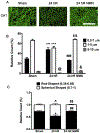
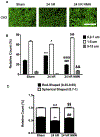
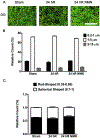
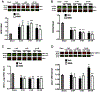
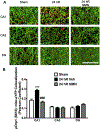
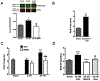
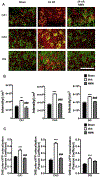
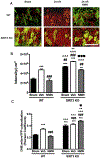
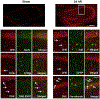
Similar articles
-
Nicotinamide mononucleotide alters mitochondrial dynamics by SIRT3-dependent mechanism in male mice.J Neurosci Res. 2019 Aug;97(8):975-990. doi: 10.1002/jnr.24397. Epub 2019 Feb 23. J Neurosci Res. 2019. PMID: 30801823 Free PMC article.
-
Nicotinamide mononucleotide inhibits post-ischemic NAD(+) degradation and dramatically ameliorates brain damage following global cerebral ischemia.Neurobiol Dis. 2016 Nov;95:102-10. doi: 10.1016/j.nbd.2016.07.018. Epub 2016 Jul 15. Neurobiol Dis. 2016. PMID: 27425894 Free PMC article.
-
Effect of nicotinamide mononucleotide on brain mitochondrial respiratory deficits in an Alzheimer's disease-relevant murine model.BMC Neurol. 2015 Mar 1;15:19. doi: 10.1186/s12883-015-0272-x. BMC Neurol. 2015. PMID: 25884176 Free PMC article.
-
Multi-_targeted Effect of Nicotinamide Mononucleotide on Brain Bioenergetic Metabolism.Neurochem Res. 2019 Oct;44(10):2280-2287. doi: 10.1007/s11064-019-02729-0. Epub 2019 Jan 19. Neurochem Res. 2019. PMID: 30661231 Review.
-
Interplay between NAD+ and acetyl‑CoA metabolism in ischemia-induced mitochondrial pathophysiology.Biochim Biophys Acta Mol Basis Dis. 2019 Aug 1;1865(8):2060-2067. doi: 10.1016/j.bbadis.2018.09.025. Epub 2018 Sep 24. Biochim Biophys Acta Mol Basis Dis. 2019. PMID: 30261291 Free PMC article. Review.
Cited by
-
Nicotinamide mononucleotides alleviated neurological impairment via anti-neuroinflammation in traumatic brain injury.Int J Med Sci. 2023 Jan 31;20(3):307-317. doi: 10.7150/ijms.80942. eCollection 2023. Int J Med Sci. 2023. PMID: 36860678 Free PMC article.
-
Nicotinamide reverses deficits in puberty-born neurons and cognitive function after maternal separation.J Neuroinflammation. 2022 Sep 21;19(1):232. doi: 10.1186/s12974-022-02591-y. J Neuroinflammation. 2022. PMID: 36131290 Free PMC article.
-
DNA damage induced PARP-1 overactivation confers paclitaxel-induced neuropathic pain by regulating mitochondrial oxidative metabolism.CNS Neurosci Ther. 2024 Sep;30(9):e70012. doi: 10.1111/cns.70012. CNS Neurosci Ther. 2024. PMID: 39215404 Free PMC article.
-
Nicotinamide Mononucleotide Supplementation Improves Mitochondrial Dysfunction and Rescues Cellular Senescence by NAD+/Sirt3 Pathway in Mesenchymal Stem Cells.Int J Mol Sci. 2022 Nov 25;23(23):14739. doi: 10.3390/ijms232314739. Int J Mol Sci. 2022. PMID: 36499074 Free PMC article.
-
Distribution of Nicotinamide Mononucleotide after Intravenous Injection in Normal and Ischemic Stroke Mice.Curr Pharm Biotechnol. 2023;24(2):299-309. doi: 10.2174/1389201023666220518113219. Curr Pharm Biotechnol. 2023. PMID: 35593333
References
Publication types
MeSH terms
Substances
Grants and funding
LinkOut - more resources
Full Text Sources