Harnessing bacterial interactions to manage infections: a review on the opportunistic pathogen Pseudomonas aeruginosa as a case example
- PMID: 31961787
- PMCID: PMC7116537
- DOI: 10.1099/jmm.0.001134
Harnessing bacterial interactions to manage infections: a review on the opportunistic pathogen Pseudomonas aeruginosa as a case example
Abstract
During infections, bacterial pathogens can engage in a variety of interactions with each other, ranging from the cooperative sharing of resources to deadly warfare. This is especially relevant in opportunistic infections, where different strains and species often co-infect the same patient and interact in the host. Here, we review the relevance of these social interactions during opportunistic infections using the human pathogen Pseudomonas aeruginosa as a case example. In particular, we discuss different types of pathogen-pathogen interactions, involving both cooperation and competition, and elaborate on how they impact virulence in multi-strain and multi-species infections. We then review evolutionary dynamics within pathogen populations during chronic infections. We particuarly discuss how local adaptation through niche separation, evolutionary successions and antagonistic co-evolution between pathogens can alter virulence and the damage inflicted on the host. Finally, we outline how studying bacterial social dynamics could be used to manage infections. We show that a deeper appreciation of bacterial evolution and ecology in the clinical context is important for understanding microbial infections and can inspire novel treatment strategies.
Keywords: Pseudomonas aeruginosa; alternative treatments; ecology and evolution; infections; polymicrobial infections; social interactions; sociomicrobiology.
Conflict of interest statement
The authors have no competing interests to declare.
Figures
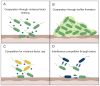
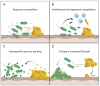
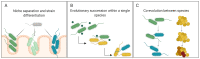
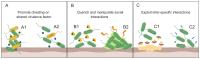
Similar articles
-
Strain Background, Species Frequency, and Environmental Conditions Are Important in Determining Pseudomonas aeruginosa and Staphylococcus aureus Population Dynamics and Species Coexistence.Appl Environ Microbiol. 2020 Sep 1;86(18):e00962-20. doi: 10.1128/AEM.00962-20. Print 2020 Sep 1. Appl Environ Microbiol. 2020. PMID: 32651205 Free PMC article.
-
Interactions between Pseudomonas aeruginosa and six opportunistic pathogens cover a broad spectrum from mutualism to antagonism.Environ Microbiol Rep. 2024 Oct;16(5):e70015. doi: 10.1111/1758-2229.70015. Environ Microbiol Rep. 2024. PMID: 39356147 Free PMC article.
-
Evolution of metabolic divergence in Pseudomonas aeruginosa during long-term infection facilitates a proto-cooperative interspecies interaction.ISME J. 2016 Jun;10(6):1323-36. doi: 10.1038/ismej.2015.220. Epub 2015 Dec 18. ISME J. 2016. PMID: 26684729 Free PMC article.
-
Interactions between Pseudomonas aeruginosa and Staphylococcus aureus during co-cultivations and polymicrobial infections.Appl Microbiol Biotechnol. 2016 Jul;100(14):6141-6148. doi: 10.1007/s00253-016-7596-3. Epub 2016 May 28. Appl Microbiol Biotechnol. 2016. PMID: 27236810 Free PMC article. Review.
-
[Pseudomonas aeruginosa: a model of choice for the study of opportunistic pathogen].Ann Fr Anesth Reanim. 2003 Jun;22(6):523-6. doi: 10.1016/s0750-7658(03)00168-0. Ann Fr Anesth Reanim. 2003. PMID: 12893377 Review. French. No abstract available.
Cited by
-
Single-Cell Imaging Reveals That Staphylococcus aureus Is Highly Competitive Against Pseudomonas aeruginosa on Surfaces.Front Cell Infect Microbiol. 2021 Aug 26;11:733991. doi: 10.3389/fcimb.2021.733991. eCollection 2021. Front Cell Infect Microbiol. 2021. PMID: 34513736 Free PMC article.
-
A Generalist Lifestyle Allows Rare Gardnerella spp. to Persist at Low Levels in the Vaginal Microbiome.Microb Ecol. 2021 Nov;82(4):1048-1060. doi: 10.1007/s00248-020-01643-1. Epub 2020 Nov 20. Microb Ecol. 2021. PMID: 33219399 Free PMC article.
-
Rapid and strain-specific resistance evolution of Staphylococcus aureus against inhibitory molecules secreted by Pseudomonas aeruginosa.mBio. 2023 Oct 31;14(5):e0315322. doi: 10.1128/mbio.03153-22. Epub 2023 Aug 30. mBio. 2023. PMID: 37646506 Free PMC article.
-
Drosophila melanogaster Systemic Infection Model to Study Altered Virulence during Polymicrobial Infection by Aeromonas.Pathogens. 2023 Mar 2;12(3):405. doi: 10.3390/pathogens12030405. Pathogens. 2023. PMID: 36986327 Free PMC article.
-
Combining antibiotics with antivirulence compounds can have synergistic effects and reverse selection for antibiotic resistance in Pseudomonas aeruginosa.PLoS Biol. 2020 Aug 18;18(8):e3000805. doi: 10.1371/journal.pbio.3000805. eCollection 2020 Aug. PLoS Biol. 2020. PMID: 32810152 Free PMC article.
References
Publication types
MeSH terms
Grants and funding
LinkOut - more resources
Full Text Sources