Design and Simulation of an Integrated Centrifugal Microfluidic Device for CTCs Separation and Cell Lysis
- PMID: 32698447
- PMCID: PMC7407509
- DOI: 10.3390/mi11070699
Design and Simulation of an Integrated Centrifugal Microfluidic Device for CTCs Separation and Cell Lysis
Abstract
Separation of circulating tumor cells (CTCs) from blood samples and subsequent DNA extraction from these cells play a crucial role in cancer research and drug discovery. Microfluidics is a versatile technology that has been applied to create niche solutions to biomedical applications, such as cell separation and mixing, droplet generation, bioprinting, and organs on a chip. Centrifugal microfluidic biochips created on compact disks show great potential in processing biological samples for point of care diagnostics. This study investigates the design and numerical simulation of an integrated microfluidic device, including a cell separation unit for isolating CTCs from a blood sample and a micromixer unit for cell lysis on a rotating disk platform. For this purpose, an inertial microfluidic device was designed for the separation of _target cells by using contraction-expansion microchannel arrays. Additionally, a micromixer was incorporated to mix separated _target cells with the cell lysis chemical reagent to dissolve their membranes to facilitate further assays. Our numerical simulation approach was validated for both cell separation and micromixer units and corroborates existing experimental results. In the first compartment of the proposed device (cell separation unit), several simulations were performed at different angular velocities from 500 rpm to 3000 rpm to find the optimum angular velocity for maximum separation efficiency. By using the proposed inertial separation approach, CTCs, were successfully separated from white blood cells (WBCs) with high efficiency (~90%) at an angular velocity of 2000 rpm. Furthermore, a serpentine channel with rectangular obstacles was designed to achieve a highly efficient micromixer unit with high mixing quality (~98%) for isolated CTCs lysis at 2000 rpm.
Keywords: cell lysis; cell separation; circulating tumor cells; microfluidics; micromixer.
Conflict of interest statement
The authors declare no conflict of interest.
Figures
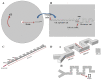
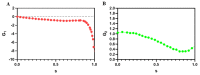
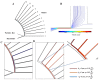
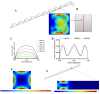
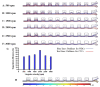
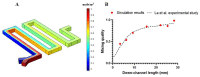
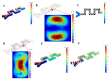
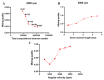
Similar articles
-
Numerical Study of a Centrifugal Platform for the Inertial Separation of Circulating Tumor Cells Using Contraction-Expansion Array Microchannels.Arch Razi Inst. 2022 Apr 30;77(2):647-660. doi: 10.22092/ARI.2022.357477.2046. eCollection 2022 Apr. Arch Razi Inst. 2022. PMID: 36284940 Free PMC article.
-
An experimental study of centrifugal microfluidic platforms for magnetic-inertial separation of circulating tumor cells using contraction-expansion and zigzag arrays.J Chromatogr A. 2023 Sep 13;1706:464249. doi: 10.1016/j.chroma.2023.464249. Epub 2023 Jul 29. J Chromatogr A. 2023. PMID: 37531849
-
A Novel Size-Based Centrifugal Microfluidic Design to Enrich and Magnetically Isolate Circulating Tumor Cells from Blood Cells through Biocompatible Magnetite-Arginine Nanoparticles.Sensors (Basel). 2024 Sep 18;24(18):6031. doi: 10.3390/s24186031. Sensors (Basel). 2024. PMID: 39338775 Free PMC article.
-
Recent Developments in Inertial and Centrifugal Microfluidic Systems along with the Involved Forces for Cancer Cell Separation: A Review.Sensors (Basel). 2023 Jun 2;23(11):5300. doi: 10.3390/s23115300. Sensors (Basel). 2023. PMID: 37300027 Free PMC article. Review.
-
Recent progress of inertial microfluidic-based cell separation.Analyst. 2021 Nov 22;146(23):7070-7086. doi: 10.1039/d1an01160j. Analyst. 2021. PMID: 34761757 Review.
Cited by
-
The Effect of Non-Uniform Magnetic Field on the Efficiency of Mixing in Droplet-Based Microfluidics: A Numerical Investigation.Micromachines (Basel). 2022 Oct 2;13(10):1661. doi: 10.3390/mi13101661. Micromachines (Basel). 2022. PMID: 36296014 Free PMC article.
-
Parallelization of Curved Inertial Microfluidic Channels to Increase the Throughput of Simultaneous Microparticle Separation and Washing.Micromachines (Basel). 2024 Sep 30;15(10):1228. doi: 10.3390/mi15101228. Micromachines (Basel). 2024. PMID: 39459102 Free PMC article.
-
Novel Isolating Approaches to Circulating Tumor Cell Enrichment Based on Microfluidics: A Review.Micromachines (Basel). 2024 May 27;15(6):706. doi: 10.3390/mi15060706. Micromachines (Basel). 2024. PMID: 38930676 Free PMC article. Review.
-
Numerical study of bacteria removal from microalgae solution using an asymmetric contraction-expansion microfluidic device: A parametric analysis approach.Heliyon. 2023 Sep 21;9(10):e20380. doi: 10.1016/j.heliyon.2023.e20380. eCollection 2023 Oct. Heliyon. 2023. PMID: 37780775 Free PMC article.
-
Numerical Study of a Centrifugal Platform for the Inertial Separation of Circulating Tumor Cells Using Contraction-Expansion Array Microchannels.Arch Razi Inst. 2022 Apr 30;77(2):647-660. doi: 10.22092/ARI.2022.357477.2046. eCollection 2022 Apr. Arch Razi Inst. 2022. PMID: 36284940 Free PMC article.
References
-
- Cohen S.J., Punt C.J.A., Iannotti N., Saidman B.H., Sabbath K.D., Gabrail N.Y., Picus J., Morse M., Mitchell E., Miller M.C., et al. Relationship of Circulating Tumor Cells to Tumor Response, Progression-Free Survival, and Overall Survival in Patients With Metastatic Colorectal Cancer. J. Clin. Oncol. 2008;26:3213–3221. doi: 10.1200/JCO.2007.15.8923. - DOI - PubMed
LinkOut - more resources
Full Text Sources