Viral gene drive in herpesviruses
- PMID: 32985507
- PMCID: PMC7522973
- DOI: 10.1038/s41467-020-18678-0
Viral gene drive in herpesviruses
Abstract
Gene drives are genetic modifications designed to propagate in a population with high efficiency. Current gene drive strategies rely on sexual reproduction and are thought to be restricted to sexual organisms. Here, we report on a gene drive system that allows the spread of an engineered trait in populations of DNA viruses and, in particular, herpesviruses. We describe the successful transmission of a gene drive sequence between distinct strains of human cytomegalovirus (human herpesvirus 5) and show that gene drive viruses can efficiently _target and replace wildtype populations in cell culture experiments. Moreover, by _targeting sequences necessary for viral replication, our results indicate that a viral gene drive can be used as a strategy to suppress a viral infection. Taken together, this work offers a proof of principle for the design of a gene drive in viruses.
Conflict of interest statement
A patent application describing the use of a gene drive in DNA viruses has been filed by the Buck Institute for Research on Aging (Application number PCT/US2019/034205, pending, inventor: M.W.). E.V. declares no competing interests.
Figures
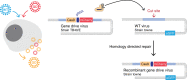
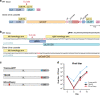
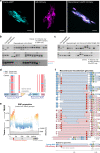
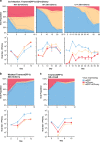
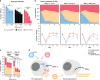
Similar articles
-
_targeting Conserved Sequences Circumvents the Evolution of Resistance in a Viral Gene Drive against Human Cytomegalovirus.J Virol. 2021 Jul 12;95(15):e0080221. doi: 10.1128/JVI.00802-21. Epub 2021 Jul 12. J Virol. 2021. PMID: 34011551 Free PMC article.
-
Viral gene drive spread during herpes simplex virus 1 infection in mice.Nat Commun. 2024 Sep 17;15(1):8161. doi: 10.1038/s41467-024-52395-2. Nat Commun. 2024. PMID: 39289368 Free PMC article.
-
CRISPR/Cas9-Mediated Genome Editing of Herpesviruses Limits Productive and Latent Infections.PLoS Pathog. 2016 Jun 30;12(6):e1005701. doi: 10.1371/journal.ppat.1005701. eCollection 2016 Jun. PLoS Pathog. 2016. PMID: 27362483 Free PMC article.
-
Human herpesviruses: a consideration of the latent state.Microbiol Rev. 1989 Sep;53(3):318-32. doi: 10.1128/mr.53.3.318-332.1989. Microbiol Rev. 1989. PMID: 2552271 Free PMC article. Review. No abstract available.
-
Potential Application of the CRISPR/Cas9 System against Herpesvirus Infections.Viruses. 2018 May 29;10(6):291. doi: 10.3390/v10060291. Viruses. 2018. PMID: 29844277 Free PMC article. Review.
Cited by
-
Next-generation CRISPR gene-drive systems using Cas12a nuclease.Nat Commun. 2023 Oct 12;14(1):6388. doi: 10.1038/s41467-023-42183-9. Nat Commun. 2023. PMID: 37821497 Free PMC article.
-
Analysis of a Cas12a-based gene-drive system in budding yeast.Access Microbiol. 2021 Dec 17;3(12):000301. doi: 10.1099/acmi.0.000301. eCollection 2021. Access Microbiol. 2021. PMID: 35024561 Free PMC article.
-
_targeting Conserved Sequences Circumvents the Evolution of Resistance in a Viral Gene Drive against Human Cytomegalovirus.J Virol. 2021 Jul 12;95(15):e0080221. doi: 10.1128/JVI.00802-21. Epub 2021 Jul 12. J Virol. 2021. PMID: 34011551 Free PMC article.
-
A Two-tiered functional screen identifies herpesviral transcriptional modifiers and their essential domains.PLoS Pathog. 2022 Jan 18;18(1):e1010236. doi: 10.1371/journal.ppat.1010236. eCollection 2022 Jan. PLoS Pathog. 2022. PMID: 35041709 Free PMC article.
-
Viral gene drive spread during herpes simplex virus 1 infection in mice.Nat Commun. 2024 Sep 17;15(1):8161. doi: 10.1038/s41467-024-52395-2. Nat Commun. 2024. PMID: 39289368 Free PMC article.
References
-
- Burrell, C. J. et al. Herpesviruses. In Fenner and White’s Medical Virology,(eds Burrell, C. J., Howard, C. R. & Murphy, F. A.) Ch. 17, 237–261 (Elsevier, 2017).
Publication types
MeSH terms
Substances
LinkOut - more resources
Full Text Sources