A novel dual Ca2+ sensor system regulates Ca2+-dependent neurotransmitter release
- PMID: 33570571
- PMCID: PMC7883739
- DOI: 10.1083/jcb.202008121
A novel dual Ca2+ sensor system regulates Ca2+-dependent neurotransmitter release
Abstract
Ca2+-dependent neurotransmitter release requires synaptotagmins as Ca2+ sensors to trigger synaptic vesicle (SV) exocytosis via binding of their tandem C2 domains-C2A and C2B-to Ca2+. We have previously demonstrated that SNT-1, a mouse synaptotagmin-1 (Syt1) homologue, functions as the fast Ca2+ sensor in Caenorhabditis elegans. Here, we report a new Ca2+ sensor, SNT-3, which triggers delayed Ca2+-dependent neurotransmitter release. snt-1;snt-3 double mutants abolish evoked synaptic transmission, demonstrating that C. elegans NMJs use a dual Ca2+ sensor system. SNT-3 possesses canonical aspartate residues in both C2 domains, but lacks an N-terminal transmembrane (TM) domain. Biochemical evidence demonstrates that SNT-3 binds both Ca2+ and the plasma membrane. Functional analysis shows that SNT-3 is activated when SNT-1 function is impaired, triggering SV release that is loosely coupled to Ca2+ entry. Compared with SNT-1, which is tethered to SVs, SNT-3 is not associated with SV. Eliminating the SV tethering of SNT-1 by removing the TM domain or the whole N terminus rescues fast release kinetics, demonstrating that cytoplasmic SNT-1 is still functional and triggers fast neurotransmitter release, but also exhibits decreased evoked amplitude and release probability. These results suggest that the fast and slow properties of SV release are determined by the intrinsically different C2 domains in SNT-1 and SNT-3, rather than their N-termini-mediated membrane tethering. Our findings therefore reveal a novel dual Ca2+ sensor system in C. elegans and provide significant insights into Ca2+-regulated exocytosis.
© 2021 Li et al.
Figures
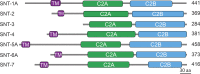
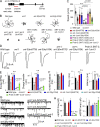
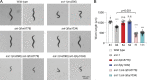
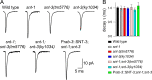
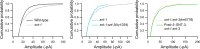
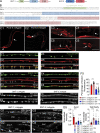
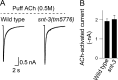
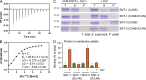
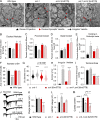
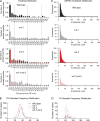
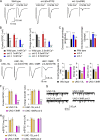
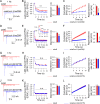
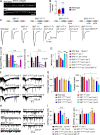
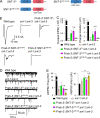
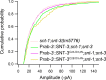
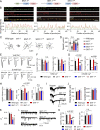
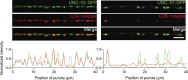
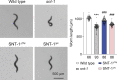
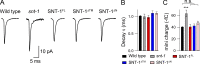
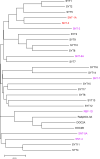
Comment in
-
Evolutionary diversity of the dual Ca2+ sensor system for neurotransmitter release.Cell Calcium. 2021 Jun;96:102402. doi: 10.1016/j.ceca.2021.102402. Epub 2021 Mar 26. Cell Calcium. 2021. PMID: 33813181
Similar articles
-
SNT-1 Functions as the Ca2+ Sensor for Tonic and Evoked Neurotransmitter Release in Caenorhabditis Elegans.J Neurosci. 2018 Jun 6;38(23):5313-5324. doi: 10.1523/JNEUROSCI.3097-17.2018. Epub 2018 May 14. J Neurosci. 2018. PMID: 29760174 Free PMC article.
-
The C2A domain of synaptotagmin is an essential component of the calcium sensor for synaptic transmission.PLoS One. 2020 Feb 7;15(2):e0228348. doi: 10.1371/journal.pone.0228348. eCollection 2020. PLoS One. 2020. PMID: 32032373 Free PMC article.
-
Polybasic Patches in Both C2 Domains of Synaptotagmin-1 Are Required for Evoked Neurotransmitter Release.J Neurosci. 2022 Jul 27;42(30):5816-5829. doi: 10.1523/JNEUROSCI.1385-21.2022. Epub 2022 Jun 14. J Neurosci. 2022. PMID: 35701163 Free PMC article.
-
Synaptotagmin I, a Ca2+ sensor for neurotransmitter release.Trends Neurosci. 2003 Aug;26(8):413-22. doi: 10.1016/S0166-2236(03)00195-4. Trends Neurosci. 2003. PMID: 12900172 Review.
-
Synaptotagmin in Ca2+ -dependent exocytosis: dynamic action in a flash.Neuron. 2003 May 22;38(4):521-4. doi: 10.1016/s0896-6273(03)00290-3. Neuron. 2003. PMID: 12765604 Review.
Cited by
-
Calcium Sensors of Neurotransmitter Release.Adv Neurobiol. 2023;33:119-138. doi: 10.1007/978-3-031-34229-5_5. Adv Neurobiol. 2023. PMID: 37615865
-
Presynaptic neurons self-tune by inversely coupling neurotransmitter release with the abundance of CaV2 voltage-gated Ca2+ channels.Proc Natl Acad Sci U S A. 2024 Aug 27;121(35):e2404969121. doi: 10.1073/pnas.2404969121. Epub 2024 Aug 22. Proc Natl Acad Sci U S A. 2024. PMID: 39172783
-
Synaptotagmins 3 and 7 mediate the majority of asynchronous release from synapses in the cerebellum and hippocampus.Cell Rep. 2024 Aug 27;43(8):114595. doi: 10.1016/j.celrep.2024.114595. Epub 2024 Aug 6. Cell Rep. 2024. PMID: 39116209 Free PMC article.
-
Mechanisms of Synaptic Vesicle Exo- and Endocytosis.Biomedicines. 2022 Jul 4;10(7):1593. doi: 10.3390/biomedicines10071593. Biomedicines. 2022. PMID: 35884898 Free PMC article. Review.
-
Protocols for electrophysiological recordings and electron microscopy at C. elegans neuromuscular junction.STAR Protoc. 2021 Aug 13;2(3):100749. doi: 10.1016/j.xpro.2021.100749. eCollection 2021 Sep 17. STAR Protoc. 2021. PMID: 34430921 Free PMC article.
References
Publication types
MeSH terms
Substances
Grants and funding
LinkOut - more resources
Full Text Sources
Other Literature Sources
Miscellaneous