Interfaces between cellular responses to DNA damage and cancer immunotherapy
- PMID: 33888558
- PMCID: PMC8091970
- DOI: 10.1101/gad.348314.121
Interfaces between cellular responses to DNA damage and cancer immunotherapy
Abstract
The DNA damage response (DDR) fulfils essential roles to preserve genome integrity. _targeting the DDR in tumors has had remarkable success over the last decade, exemplified by the licensing of PARP inhibitors for cancer therapy. Recent studies suggest that the application of DDR inhibitors impacts on cellular innate and adaptive immune responses, wherein key DNA repair factors have roles in limiting chronic inflammatory signaling. Antitumor immunity plays an emerging part in cancer therapy, and extensive efforts have led to the development of immune checkpoint inhibitors overcoming immune suppressive signals in tumors. Here, we review the current understanding of the molecular mechanisms underlying DNA damage-triggered immune responses, including cytosolic DNA sensing via the cGAS/STING pathway. We highlight the implications of DDR components for therapeutic outcomes of immune checkpoint inhibitors or their use as biomarkers. Finally, we discuss the rationale for novel combinations of DDR inhibitors with antagonists of immune checkpoints and current hindrances limiting their broader therapeutic applications.
Keywords: DNA damage response; DNA repair; PARP inhibitors; PD-1; PD-L1; STING; cGAS; immunotherapy.
© 2021 Pilger et al.; Published by Cold Spring Harbor Laboratory Press.
Figures
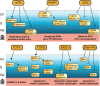
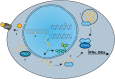
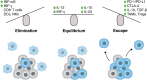
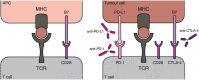
Similar articles
-
The complementarity of DDR, nucleic acids and anti-tumour immunity.Nature. 2023 Jul;619(7970):475-486. doi: 10.1038/s41586-023-06069-6. Epub 2023 Jul 19. Nature. 2023. PMID: 37468584 Review.
-
Function and Molecular Mechanism of the DNA Damage Response in Immunity and Cancer Immunotherapy.Front Immunol. 2021 Dec 14;12:797880. doi: 10.3389/fimmu.2021.797880. eCollection 2021. Front Immunol. 2021. PMID: 34970273 Free PMC article. Review.
-
_targeting DNA Repair Response Promotes Immunotherapy in Ovarian Cancer: Rationale and Clinical Application.Front Immunol. 2021 Oct 12;12:661115. doi: 10.3389/fimmu.2021.661115. eCollection 2021. Front Immunol. 2021. PMID: 34712221 Free PMC article. Review.
-
_targeting DNA Repair in Cancer: Beyond PARP Inhibitors.Cancer Discov. 2017 Jan;7(1):20-37. doi: 10.1158/2159-8290.CD-16-0860. Epub 2016 Dec 21. Cancer Discov. 2017. PMID: 28003236 Free PMC article. Review.
-
_targeting DNA damage response and repair genes to enhance anticancer immunotherapy: rationale and clinical implication.Future Oncol. 2020 Aug;16(23):1751-1766. doi: 10.2217/fon-2020-0215. Epub 2020 Jun 15. Future Oncol. 2020. PMID: 32539551 Review.
Cited by
-
_targeting DNA Damage Repair and Immune Checkpoint Proteins for Optimizing the Treatment of Endometrial Cancer.Pharmaceutics. 2023 Aug 30;15(9):2241. doi: 10.3390/pharmaceutics15092241. Pharmaceutics. 2023. PMID: 37765210 Free PMC article. Review.
-
BOP1 Used as a Novel Prognostic Marker and Correlated with Tumor Microenvironment in Pan-Cancer.J Oncol. 2021 Sep 22;2021:3603030. doi: 10.1155/2021/3603030. eCollection 2021. J Oncol. 2021. PMID: 34603446 Free PMC article.
-
MUC1-C Dictates PBRM1-Mediated Chronic Induction of Interferon Signaling, DNA Damage Resistance, and Immunosuppression in Triple-Negative Breast Cancer.Mol Cancer Res. 2023 Mar 1;21(3):274-289. doi: 10.1158/1541-7786.MCR-22-0772. Mol Cancer Res. 2023. PMID: 36445328 Free PMC article.
-
DNA-PK Inhibition and Radiation Promote Antitumoral Immunity through RNA Polymerase III in Pancreatic Cancer.Mol Cancer Res. 2022 Jul 6;20(7):1137-1150. doi: 10.1158/1541-7786.MCR-21-0725. Mol Cancer Res. 2022. PMID: 35348737 Free PMC article.
-
_targeting replication stress in cancer therapy.Nat Rev Drug Discov. 2023 Jan;22(1):38-58. doi: 10.1038/s41573-022-00558-5. Epub 2022 Oct 6. Nat Rev Drug Discov. 2023. PMID: 36202931 Free PMC article. Review.
References
Publication types
MeSH terms
Substances
Grants and funding
LinkOut - more resources
Full Text Sources
Other Literature Sources
Research Materials