KCNQ Channels in the Mesolimbic Reward Circuit Regulate Nociception in Chronic Pain in Mice
- PMID: 33900570
- PMCID: PMC8099961
- DOI: 10.1007/s12264-021-00668-x
KCNQ Channels in the Mesolimbic Reward Circuit Regulate Nociception in Chronic Pain in Mice
Abstract
Mesocorticolimbic dopaminergic (DA) neurons have been implicated in regulating nociception in chronic pain, yet the mechanisms are barely understood. Here, we found that chronic constructive injury (CCI) in mice increased the firing activity and decreased the KCNQ channel-mediated M-currents in ventral tegmental area (VTA) DA neurons projecting to the nucleus accumbens (NAc). Chemogenetic inhibition of the VTA-to-NAc DA neurons alleviated CCI-induced thermal nociception. Opposite changes in the firing activity and M-currents were recorded in VTA DA neurons projecting to the medial prefrontal cortex (mPFC) but did not affect nociception. In addition, intra-VTA injection of retigabine, a KCNQ opener, while reversing the changes of the VTA-to-NAc DA neurons, alleviated CCI-induced nociception, and this was abolished by injecting exogenous BDNF into the NAc. Taken together, these findings highlight a vital role of KCNQ channel-mediated modulation of mesolimbic DA activity in regulating thermal nociception in the chronic pain state.
Keywords: Brain-derived neurotrophic factor; Chronic neuropathic pain; KCNQ; Mesocorticolimbic system; Nociception; Retigabine; Ventral tegmental area.
Conflict of interest statement
The authors declare no competing interests.
Figures
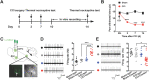
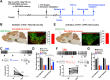
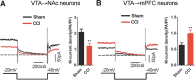
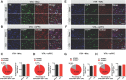
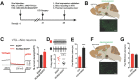
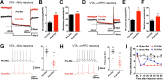
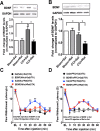
Similar articles
-
Brain-Derived Neurotrophic Factor in the Mesolimbic Reward Circuitry Mediates Nociception in Chronic Neuropathic Pain.Biol Psychiatry. 2017 Oct 15;82(8):608-618. doi: 10.1016/j.biopsych.2017.02.1180. Epub 2017 Mar 1. Biol Psychiatry. 2017. PMID: 28390647 Free PMC article.
-
Brain-derived neurotrophic factor-mediated projection-specific regulation of depressive-like and nociceptive behaviors in the mesolimbic reward circuitry.Pain. 2018 Jan;159(1):175. doi: 10.1097/j.pain.0000000000001083. Pain. 2018. PMID: 29076919 Free PMC article.
-
Projection-specific dopamine neurons in the ventral tegmental area participated in morphine-induced hyperalgesia and anti-nociceptive tolerance in male mice.J Psychopharmacol. 2021 May;35(5):591-605. doi: 10.1177/0269881120985183. Epub 2021 Mar 21. J Psychopharmacol. 2021. PMID: 33749357
-
A new aspect of chronic pain as a lifestyle-related disease.Neurobiol Pain. 2017 Apr 17;1:6-15. doi: 10.1016/j.ynpai.2017.04.003. eCollection 2017 Jan-Jul. Neurobiol Pain. 2017. PMID: 31194049 Free PMC article. Review.
-
Stress-induced plasticity and functioning of ventral tegmental dopamine neurons.Neurosci Biobehav Rev. 2020 Jan;108:48-77. doi: 10.1016/j.neubiorev.2019.10.015. Epub 2019 Oct 27. Neurosci Biobehav Rev. 2020. PMID: 31666179 Review.
Cited by
-
Autism-associated mutations in KV7 channels induce gating pore current.Proc Natl Acad Sci U S A. 2021 Nov 9;118(45):e2112666118. doi: 10.1073/pnas.2112666118. Proc Natl Acad Sci U S A. 2021. PMID: 34728568 Free PMC article.
-
Dynamic Changes of the Infralimbic Cortex and Its Regulation of the Prelimbic Cortex in Rats with Chronic Inflammatory Pain.Neurosci Bull. 2024 Jul;40(7):872-886. doi: 10.1007/s12264-023-01159-x. Epub 2024 Jan 5. Neurosci Bull. 2024. PMID: 38180711 Free PMC article.
-
Behavior of KCNQ Channels in Neural Plasticity and Motor Disorders.Membranes (Basel). 2022 May 6;12(5):499. doi: 10.3390/membranes12050499. Membranes (Basel). 2022. PMID: 35629827 Free PMC article. Review.
-
Disinhibition of Mesolimbic Dopamine Circuit by the Lateral Hypothalamus Regulates Pain Sensation.J Neurosci. 2023 Jun 14;43(24):4525-4540. doi: 10.1523/JNEUROSCI.2298-22.2023. Epub 2023 May 15. J Neurosci. 2023. PMID: 37188517 Free PMC article.
-
The Formation and Function of the VTA Dopamine System.Int J Mol Sci. 2024 Mar 30;25(7):3875. doi: 10.3390/ijms25073875. Int J Mol Sci. 2024. PMID: 38612683 Free PMC article. Review.
References
MeSH terms
LinkOut - more resources
Full Text Sources
Medical