Ultrasound-assisted production and optimization of mini-emulsions in a microfluidic chip in continuous-flow
- PMID: 33915482
- PMCID: PMC8093933
- DOI: 10.1016/j.ultsonch.2021.105556
Ultrasound-assisted production and optimization of mini-emulsions in a microfluidic chip in continuous-flow
Abstract
The use of ultrasound to generate mini-emulsions (50 nm to 1 μm in diameter) and nanoemulsions (mean droplet diameter < 200 nm) is of great relevance in drug delivery, particle synthesis and cosmetic and food industries. Therefore, it is desirable to develop new strategies to obtain new formulations faster and with less reagent consumption. Here, we present a polydimethylsiloxane (PDMS)-based microfluidic device that generates oil-in-water or water-in-oil mini-emulsions in continuous flow employing ultrasound as the driving force. A Langevin piezoelectric attached to the same glass slide as the microdevice provides enough power to create mini-emulsions in a single cycle and without reagents pre-homogenization. By introducing independently four different fluids into the microfluidic platform, it is possible to gradually modify the composition of oil, water and two different surfactants, to determine the most favorable formulation for minimizing droplet diameter and polydispersity, employing less than 500 µL of reagents. It was found that cavitation bubbles are the most important mechanism underlying emulsions formation in the microchannels and that degassing of the aqueous phase before its introduction to the device can be an important factor for reduction of droplet polydispersity. This idea is demonstrated by synthetizing solid polymeric particles with a narrow size distribution starting from a mini-emulsion produced by the device.
Keywords: High-power ultrasound; In-line emulsification; Microfluidics; Polymeric particles synthesis; Ultrasonic emulsification; W/o and o/w emulsions.
Copyright © 2021 The Authors. Published by Elsevier B.V. All rights reserved.
Conflict of interest statement
The authors declare that they have no known competing financial interests or personal relationships that could have appeared to influence the work reported in this paper.
Figures
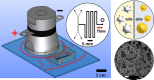
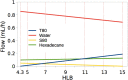
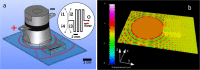
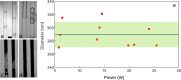
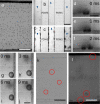
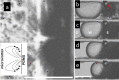
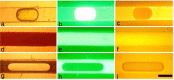
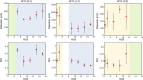
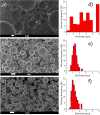
Similar articles
-
A versatile and robust microfluidic device for capillary-sized simple or multiple emulsions production.Biomed Microdevices. 2018 Oct 30;20(4):94. doi: 10.1007/s10544-018-0340-2. Biomed Microdevices. 2018. PMID: 30377821
-
Impact of osmotic pressure and gelling in the generation of highly stable single core water-in-oil-in-water (W/O/W) nano multiple emulsions of aspirin assisted by two-stage ultrasonic cavitational emulsification.Colloids Surf B Biointerfaces. 2013 Feb 1;102:653-8. doi: 10.1016/j.colsurfb.2012.08.036. Epub 2012 Sep 8. Colloids Surf B Biointerfaces. 2013. PMID: 23107943
-
Ultrasonic assisted water-in-oil emulsions encapsulating macro-molecular polysaccharide chitosan: Influence of molecular properties, emulsion viscosity and their stability.Ultrason Sonochem. 2020 Jun;64:105018. doi: 10.1016/j.ultsonch.2020.105018. Epub 2020 Feb 11. Ultrason Sonochem. 2020. PMID: 32070902
-
Formation of stable nanoemulsions by ultrasound-assisted two-step emulsification process for topical drug delivery: Effect of oil phase composition and surfactant concentration and loratadine as ripening inhibitor.Int J Pharm. 2020 Feb 25;576:118952. doi: 10.1016/j.ijpharm.2019.118952. Epub 2019 Dec 14. Int J Pharm. 2020. PMID: 31843549
-
Complex Emulsions as an Innovative Pharmaceutical Dosage form in Addressing the Issues of Multi-Drug Therapy and Polypharmacy Challenges.Pharmaceutics. 2024 May 24;16(6):707. doi: 10.3390/pharmaceutics16060707. Pharmaceutics. 2024. PMID: 38931830 Free PMC article. Review.
Cited by
-
Mechanisms of lipid oxidation in water-in-oil emulsions and oxidomics-guided discovery of _targeted protective approaches.Compr Rev Food Sci Food Saf. 2023 Jul;22(4):2678-2705. doi: 10.1111/1541-4337.13158. Epub 2023 Apr 25. Compr Rev Food Sci Food Saf. 2023. PMID: 37097053 Free PMC article. Review.
References
-
- Slomkowski S., Alemán J.V., Gilbert R.G., Hess M., Horie K., Jones R.G., Kubisa P., Meisel I., Mormann W., Penczek S., Stepto R.F.T. Terminology of polymers and polymerization processes in dispersed systems (IUPAC recommendations 2011) Pure Appl. Chem. 2011;83(2011):2229–2259. doi: 10.1351/PAC-REC-10-06-03. - DOI
-
- Jafari S., McClements D.J., editors. Nanoemulsions. Applications and Characterization, Academic Press; Formulation: 2018.
-
- Gianella A., Jarzyna P.A., Mani V., Ramachandran S., Calcagno C., Tang J., Kann B., Dijk W.J.R., Thijssen V.L., Griffioen A.W., Storm G., Fayad Z.A., Mulder W.J.M. Multifunctional Nanoemulsion Platform for Imaging Guided Therapy Evaluated in Experimental Cancer. ACS Nano. 2011;5:4422–4433. doi: 10.1021/nn103336a. - DOI - PMC - PubMed
MeSH terms
Substances
LinkOut - more resources
Full Text Sources
Other Literature Sources