Structural dynamics of SARS-CoV-2 variants: A health monitoring strategy for anticipating Covid-19 outbreaks
- PMID: 34089757
- PMCID: PMC8172274
- DOI: 10.1016/j.jinf.2021.06.001
Structural dynamics of SARS-CoV-2 variants: A health monitoring strategy for anticipating Covid-19 outbreaks
Abstract
Objectives: the Covid-19 pandemic has been marked by sudden outbreaks of SARS-CoV-2 variants harboring mutations in both the N-terminal (NTD) and receptor binding (RBD) domains of the spike protein. The goal of this study was to predict the transmissibility of SARS-CoV-2 variants from genomic sequence data.
Methods: we used a _target-based molecular modeling strategy combined with surface potential analysis of the NTD and RBD.
Results: we observed that both domains act synergistically to ensure optimal virus adhesion, which explains why most variants exhibit concomitant mutations in the RBD and in the NTD. Some mutation patterns affect the affinity of the spike protein for ACE-2. However, other patterns increase the electropositive surface of the spike, with determinant effects on the kinetics of virus adhesion to lipid raft gangliosides. Based on this new view of the structural dynamics of SARS-CoV-2 variants, we defined an index of transmissibility (T-index) calculated from kinetic and affinity parameters of coronavirus binding to host cells. The T-index is characteristic of each variant and predictive of its dissemination in animal and human populations.
Conclusions: the T-index can be used as a health monitoring strategy to anticipate future Covid-19 outbreaks due to the emergence of variants of concern.
Keywords: ACE-2; Coronavirus; Electrostatic surface potential; Ganglioside; Lipid raft; SARS-CoV-2; Virus-host interactions; receptor.
Copyright © 2021. Published by Elsevier Ltd.
Conflict of interest statement
Declaration of Competing Interest The authors declare no conflict of interest.
Figures
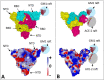
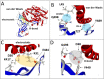
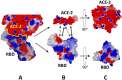
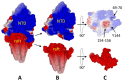
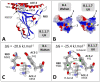
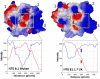
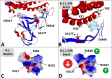
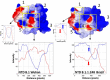
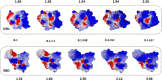
Comment in
-
A stark difference in the profiles of defective viral transcripts between SARS-CoV-2 and SARS-CoV.J Infect. 2021 Sep;83(3):381-412. doi: 10.1016/j.jinf.2021.06.020. Epub 2021 Jul 1. J Infect. 2021. PMID: 34216637 Free PMC article. No abstract available.
-
Is diffusion of SARS-CoV-2 variants of concern associated with different symptoms?J Infect. 2022 Jan;84(1):94-118. doi: 10.1016/j.jinf.2021.07.008. Epub 2021 Jul 15. J Infect. 2022. PMID: 34274361 Free PMC article. No abstract available.
Similar articles
-
V367F Mutation in SARS-CoV-2 Spike RBD Emerging during the Early Transmission Phase Enhances Viral Infectivity through Increased Human ACE2 Receptor Binding Affinity.J Virol. 2021 Jul 26;95(16):e0061721. doi: 10.1128/JVI.00617-21. Epub 2021 Jul 26. J Virol. 2021. PMID: 34105996 Free PMC article.
-
Synergistic antiviral effect of hydroxychloroquine and azithromycin in combination against SARS-CoV-2: What molecular dynamics studies of virus-host interactions reveal.Int J Antimicrob Agents. 2020 Aug;56(2):106020. doi: 10.1016/j.ijantimicag.2020.106020. Epub 2020 May 13. Int J Antimicrob Agents. 2020. PMID: 32405156 Free PMC article.
-
Effects of common mutations in the SARS-CoV-2 Spike RBD and its ligand, the human ACE2 receptor on binding affinity and kinetics.Elife. 2021 Aug 26;10:e70658. doi: 10.7554/eLife.70658. Elife. 2021. PMID: 34435953 Free PMC article.
-
How Do Point Mutations Enhancing the Basic Character of the RBDs of SARS-CoV-2 Variants Affect Their Transmissibility and Infectivity Capacities?Viruses. 2022 Apr 10;14(4):783. doi: 10.3390/v14040783. Viruses. 2022. PMID: 35458513 Free PMC article. Review.
-
Surface charge changes in spike RBD mutations of SARS-CoV-2 and its variant strains alter the virus evasiveness via HSPGs: A review and mechanistic hypothesis.Front Public Health. 2022 Aug 24;10:952916. doi: 10.3389/fpubh.2022.952916. eCollection 2022. Front Public Health. 2022. PMID: 36091499 Free PMC article. Review.
Cited by
-
First cases of infection with the 21L/BA.2 Omicron variant in Marseille, France.J Med Virol. 2022 Jul;94(7):3421-3430. doi: 10.1002/jmv.27695. Epub 2022 Mar 24. J Med Virol. 2022. PMID: 35243660 Free PMC article.
-
Rapid evolution of SARS-CoV-2 challenges human defenses.Sci Rep. 2022 Apr 19;12(1):6457. doi: 10.1038/s41598-022-10097-z. Sci Rep. 2022. PMID: 35440671 Free PMC article.
-
A stark difference in the profiles of defective viral transcripts between SARS-CoV-2 and SARS-CoV.J Infect. 2021 Sep;83(3):381-412. doi: 10.1016/j.jinf.2021.06.020. Epub 2021 Jul 1. J Infect. 2021. PMID: 34216637 Free PMC article. No abstract available.
-
Limited spread of a rare spike E484K-harboring SARS-CoV-2 in Marseille, France.Arch Virol. 2022 Feb;167(2):583-589. doi: 10.1007/s00705-021-05331-4. Epub 2022 Jan 27. Arch Virol. 2022. PMID: 35083577 Free PMC article.
-
The Biological Properties of the SARS-CoV-2 Cameroon Variant Spike: An Intermediate between the Alpha and Delta Variants.Pathogens. 2022 Jul 20;11(7):814. doi: 10.3390/pathogens11070814. Pathogens. 2022. PMID: 35890058 Free PMC article.
References
-
- Volz E., Mishra S., Chand M., Barrett J.C., Johnson R., Geidelberg L., Hinsley W.R. Assessing transmissibility of SARS-CoV-2 lineage B.1.1.7 in England. Nature. 2021;593:266–269. - PubMed
-
- Tegally H., Wilkinson E., Giovanetti M., Iranzadeh A., Fonseca V., Giandhari J., Doolabh D., Pillay S., San E.J., Msomi N. Detection of a SARS-CoV-2 variant of concern in South Africa. Nature. 2021;592:438–443. - PubMed
-
- Vaidyanathan G. Coronavirus variants are spreading in India - what scientists know so far. Nature. 2021;593:321–322. - PubMed
MeSH terms
Substances
LinkOut - more resources
Full Text Sources
Other Literature Sources
Medical
Miscellaneous